Abstract
Reduced glutathione (GSH) and homologous ram seminal plasma (HSP), used as additives in cryopreserving (CP) media prior to freezing, showed conflicting results in retaining structural integrity and progressive motility in post-thawed ram spermatozoa. The aims of this research were: (1) to assess the effect of GSH and/or HSP supplementation via soybean-lecithin CP extender on cryopreserved ram spermatozoa viability, morphology and motility pattern; and (2) to assess the effect of incubation in the context of the previous aim. Quantitatively and qualitatively, homogenized and pooled ram ejaculates (N=10) were extended with one of the following extenders: control (C) – tris-based, GSH and HSP-free, experimental-1 (E1) – C + GSH 5 mM, experimental-2 (E2) – C + HSP 20 % and experimental-3 (E3) - GSH 5 mM + HSP 20 %. Following thawing, samples were taken at 0- and 3-hours from each group (n=10) and were assessed for spermatozoa viability, morphology, and motility pattern. C-0h samples yielded a spermatozoa population with low viability, altered head morphology and highly deviated motility pattern. E3-3h samples yielded spermatozoa with unaffected viability, head morphology and high progressive motility. In conclusion, E3 extender added to cryopreserved-thawed ram spermatozoa is most efficient in obtaining high viability, unaltered head morphology, and progressive motility.
Keywords: ram, ejaculates, cryopreservation, homologous seminal plasma, reduced glutathione
INTRODUCTION
Ram spermatozoa are highly susceptible to cryo-injury compared to other livestock species (
1). Cryopreservation (CP) in liquid nitrogen affects cells with regards to their morphological (plasma and acrosome membrane integrity, head and tail morphology) and functional characteristics (motility and velocity) (
1). These spermatozoa traits are reported as predicting indices for the fertilization capacity of ram ejaculates (
2,
3,
4). The seminal plasma is the primary source of spermatozoa antioxidant protection (
5) and membrane-reparative-compound utilization (
6), having paramount importance on the cryo-survival rate (
7,
8,
9). Various antioxidant (
10,
11,
12) and seminal plasma compounds (
6,
13,
14) have been used as supplements of semen extenders for ram ejaculates, reporting conflicting results in CP survival. Sperm cells in mammalian species contain a low intracellular concentration of reduced glutathione (GSH), but its abundant presence in the seminal plasma complements the ejaculate antioxidant system (
5). Laboratory handling and extension of CP ejaculates reduce their oxyradical scavenging capacity (
15). GSH is highly correlated with other glutathione-dependent enzymes (glutathione peroxidase and reductase) which have a key role in oxyradical scavenging and are most effective in retaining mitochondrial activity and integrity (
5). Scientific reports refer that 5 mM concentrations of GSH in tris-based extenders can result in maintaining higher spermatozoa motility and membrane integrity, compared to oxidized glutathione in the same concentration (
12,
16). Homologous seminal plasma (HSP) has been used as an additive in CP media and is reported to prevent cryo-injury of the plasma membrane (Pm) by inhibition of tyrosine phosphorylation (
9). HSP is attributed to retaining a higher number of viable cells with intact structural integrity (
17) and maintaining antioxidative enzymes activity on cell surfaces (
7) following CP. Barrios et al. (
6) have isolated and identified two ram seminal plasma fractions of 14- and 20 kDa (RSP-14, RSP- 20), which have been attributed for its reparative properties on cryo- injured spermatozoa by stabilizing their membrane phospholipid and cytoskeleton structures (
8). Some reports are in agreement with these findings (
18,
19,
20), others refer to detrimental effects triggered by hyper-activating components of HSP (
21,
22,
23). To our best knowledge, there is no scientific report on the combined use of supplementary GSH and HSP in CP media in an attempt to achieve higher CP success in ram spermatozoa. Therefore, the aims of this research were: (
1) To assess the effect of GSH and/or HSP supplementation via soybean-lecithin CP extender on cryopreserved ram spermatozoa viability, morphology and motility; and (
2) To assess the effect of incubation in the context of the previous aim.
MATERIAL AND METHODS
Experimental design
The pooled volume of fresh ejaculates (4 ml) was extended with control CP-extender (soybean- lecithin based, GSH and HSP free) to spermatozoa concentration of 100x106 cell/ml. The ejaculate pool was packed in plastic straws of 0.5 ml (N=40) which were automatically frozen in liquid nitrogen (Ice Cube 15M). Following thawing (38 °С, 30 sec), straws were divided into four equal batches, its contents placed in glass tubes warmed at 37 °С and extended up to 50x106 cell/ml with one of the following extenders: Batch-1 was extended with control (C) (soybean-based, GSH, and HSP free), Batch-2 with experimental-1 (E1) (C + GSH 5 mM), Batch-3 with experimental-2 (E2) (C + HSP 20 %) and Batch-4 with experimental-3 (E3) (GSH 5 mM + HSP 20 %) CP-extenders, respectively. Following this, the samples were placed in incubation conditions (37 °С, 90 % relative humidity), and were analyzed at 0- and 3-hours (N=80). Analyzed samples were categorized according to the extender and incubation time in one of the following groups: C- 0h, C-3h, E1-0h, E1-3h, E2-0h, E2-3h and E3-0h, E3-3h. Spermatozoa were analyzed for morphology (staining-Hancock 2), trajectory pattern and velocity (Computer Assisted Semen Analysis – CASA, Hamilton Thorne).
Ejaculate and seminal plasma collection; sample pooling
Fresh ejaculates were obtained from Ovchepolska Pramenka rams (N=10) by the artificial vagina method, in accordance with the EU directive 2016/63/EU on the protection of animals used for scientific purposes (
24). They were assessed for compliance with minimum qualitative criteria (
10) in order to achieve qualitative homogenization. Compliant ejaculates (eligible samples) were pooled according to cell-ejaculate volume ratio (spermatocrit), using the following estimation: Ve (%) = (S / Si) / (S / Si) x 100, where ‘Ve’ is the ejaculate partial volume in the pool, ‘S’ is the mean spermatocrit value of individual ejaculates and ‘Si’ is the individual spermatocrit value. The procedure of pooling was performed in order to achieve quantitative homogenization.
Homologous seminal plasma (HSP) was obtained from fresh ram ejaculates (N=10), which were processed in two-phase centrifugations (I – 2000 x g, 4 °C, 20 min.; II- 2500 x g, 4 °C, 30 min), pooled and frozen at -20 °С until being used (
18).
Extenders preparation
A soybean-lecithin based CP extender (animal- protein- and GSH-free) was used for the preparation of control and experimental extenders. Three aliquots of sterile water were prepared: 1) GSH- free, 2) GSH supplemented with (5.0 mM), and 3) GSH supplemented with 7.5 mM (GSH – γ-L- Glutamyl-L- cysteinyl-glycine; cell culture tested, ≥98.0 %, powder; Sigma Aldrich®). They were added in 1:5 ratio to the CP extender as follows: the first sterile water aliquot was used for the preparation of C and E2 extenders, whereas the second and third for the preparation of E1 and E3 extenders, respectively. HSP was thawed at room temperature and immediately supplemented to the E2 and E3 extenders. Following these procedures, E1, E2 and E3 extenders contained either 5 mM of GSH, 20 % of HSP, or both, respectively. Extenders specific weight at t=21 °C was adjusted at 1.045.
Cryopreservation, thawing and post-thawing incubation of samples
Samples were packed in plastic straws (0.5 ml) and were subjected to an automated freezing protocol in liquid nitrogen (Ice Cube 15M), described in detail elsewhere (20). The plastic straws were thawed in a water bath at 38 °С (30 sec) and their content was transferred to prewarmed glass tubes at 37 °С. Following thawing, samples were incubated up to 3 hours at 37 °С and 90 % humidity.
Spermatozoa head morphology, structural integrity, trajectory pattern and velocity assessment
Structural integrity status of spermatozoa was assessed by supravital staining - Hancock 2 (25). Each replicate was sampled in 10 μl aliquot and mixed with an equal volume of Hancock-2 stain. Following 30 sec incubation (30 °C), a droplet was placed on a microscope slide (25 mm x 75 mm) and was smeared using another slide at a 30° angle. Following drying, smears were analyzed on a light- microscope at x1000 magnification (Nikon, USA). Spermatozoa structural integrity was assessed according to plasma- (Pm), acrosome membrane integrity (Ac) and overall morphology (Mo), being categorized according to their status (unaffected or affected, +/-) in the following categories: I-a (Pm+, Ac+, Mo+), I-b (Pm+, Ac-, Mo+), II-a (Pm-, Ac+, Mo+), II-b (Pm-, Ac-, Mo+) and III (Pm-, Ac-, Mo-). Spermatozoa compliant with the criteria for the first two categories were considered as viable spermatozoa.
CASA parameters were acquired using the following system settings (Hamilton Thorne v.12): frames per second – 60 Hz, number of frames – x30; minimum contrast threshold for cell detection – 60; minimum cell size threshold – 5 pix; high velocity cells threshold – VAP ≥75 μ/s; static cells threshold – VAP ≤21.9 μ/s и VSL ≤6 μ/s. Each sample was placed on a microscope slide (25 mm x 75 mm) and covered with a coverslip (18 mm x 18 mm). According to the recommendations of the WHO guidebook for semen analysis (26), 6.5 μl volume of sample was placed under the slip creating a chamber depth of 20 μm. Scanning was performed on the same start-end position of each slide (5-10 mm), including 500±50 cells for each sample. Head elongation index (EL) was expressed as percentile values of the spermatozoa head width- to-length ratio. According to its value, cells were Trajectory velocity was assessed by curvilinear track velocity (VCL, μ/s), and according to its value, cells were categorized as VCL1 (0-150 μ/s); VCL2 (151-250 μ/s); VCL3 (251-450 μ/s). Beat cross frequency (BCF, Hz) was expressed as a number of interceptions of the sperm head with the average path trajectory (Hz). Cells were categorized in three value range categories: BCF1 (0-18 Hz); BCF2 (19-30 Hz); BCF3 (31-60 Hz). Trajectory linearity (LIN, %) was assessed according to the ratio of a straight line- (VSL) and curvilinear track velocity (VCL), expressed in percentile values. Cells were categorized in three value range categories: LIN1 (0-30 %); LIN2 (31-50 %); LIN3 (51-100 %). The amplitude of lateral head displacement (ALH, μm) was assessed on CASA and was expressed as an average distance of head deviation from the curvilinear trajectory, expressed in micrometers (μm). Cells were categorized in three value range categories: ALH1 (0-6 μm); ALH2 (7-12 μm); ALH3 (13-30 μm).
The threshold values for pre-capacitation motility of ram spermatozoa for 60 Hz CASA assessment were: VCL > 250 μm/s, VSL ≤ 100 μm/s, LIN ≤ 30%, and ALH > 9 μm (
27). Spermatozoa categorized in the value range categories for the aforementioned CASA indices were considered to have non- hyperactivated motility patterns and linear trajectory. Positive effects of the factors were acknowledged for higher-than-expected number of spermatozoa (HEF) in VIAB1, EL1/EL2, VCL1/VCL2, ALH1/ALH2, LIN2/LIN3, and VEL1/VEL2, or lower-than-expected number of spermatozoa (LEF) for the remaining categories in each parameter. Negative effects of the factors were attributed to inverse spermatozoa distribution in the categories (
27).
Statistical analysis
A priori analysis was performed (G*Power v.3.1) to acquire critical χ2 value according to the degrees of freedom. Data were organized in contingency tables of 8x5 for structural integrity, and 8x3 for CASA indices (EL, VCL, ALH, BCF and LIN), respective to the number of categories. The non- parametric structure of the data was processed with Pearson’s chi-square of independence analysis (χ2 test). Additionally, adjusted residuals (±z) and its relative contribution indexes (RCI) were used for detecting the relation of each table- cell to the χ2 value (
28). Results were interpreted by identifying z values above/below the critical categorized in three index range categories: adjusted residual value (z ), i.e. higher/lower than EL1 (0-30 %), EL2 (31-50 %) and EL3 (51-100%). expected frequencies of spermatozoa (HEF/LEF), which were marked with a significant effect on overall differences (p <0.01, 99 % confidence) and were then evaluated by their respective RCI values.
RESULTS
The following a priori attributes were taken into consideration for the 8x5 (d.f. 42) and 8x3 (d.f. 14) χ2 test: non-centrality parameter (λ)=62.75 and 44.25, critical χ2 value=66.21 and 29.14, total sample size N)=251 and 177, respectively (Cohen’s effect size=0.5, target power (w)=0.99, ‘α’ probability level <0.01). Estimated χ2 values reported in the following text were significantly higher than the corresponding critical values, with the respective degrees of freedom. The results were considered as highly statistically significant (p <0.00001) and with a high confidence level > 99 %. Estimated z values for d.f. 42 and 14 were ±3.23 and ±3.08, respectively. The frequency distribution ( f ) of spermatozoa in parameter categories is reported as the number of cells with its respective RCI value, corresponding to a significant ±z value.
Spermatozoa structural integrity and head morphometry indices
More than half of the analyzed spermatozoa for structural integrity were distributed in viable categories (Ia, 42.84 % and Ib, 11.69 %), while remaining in affected structural integrity categories (Iia 22.53 %, Iib 19.73 % and III3.21 %). Frequency distribution of spermatozoa (N=8,343) is shown in
Table 1. The highest positive distance (z) from the omnibus chi-square value (χ2=1,228.54) was observed for E2-3h in Ib ( f=31.46 %, RCI=0.29), as well as the highest negative for the Ia spermatozoa category ( f=22.05 %, RCI=0.09). Similar RCIs for positive ( f=24.67 %, RCI=0.12) and negative ( f=26.38 %, RCI=0.05) z values in the same categories was visible for E3-3h. E2-0h had positive z in Ia ( f=59.20 %, RCI=0.05) and negative in Ib ( f=3.51 %, RCI=0.05). Similarly, E3-0h, had positive ( f=57.77 %, RCI=0.04) and negative ( f=3.53 %, RCI=0.05) z in the respective categories. C-0h had solely affected spermatozoa from category III with positive z ( f=7.81 %, RCI=0.06), while negative was observed in Ib ( f=2.82 %, RCI=0.06). E1-0h had minor RCIs with positive ( f=48.35 %, RCI=0.01) in Ia and negative z in Ib ( f=5.04 %, RCI=0.03).
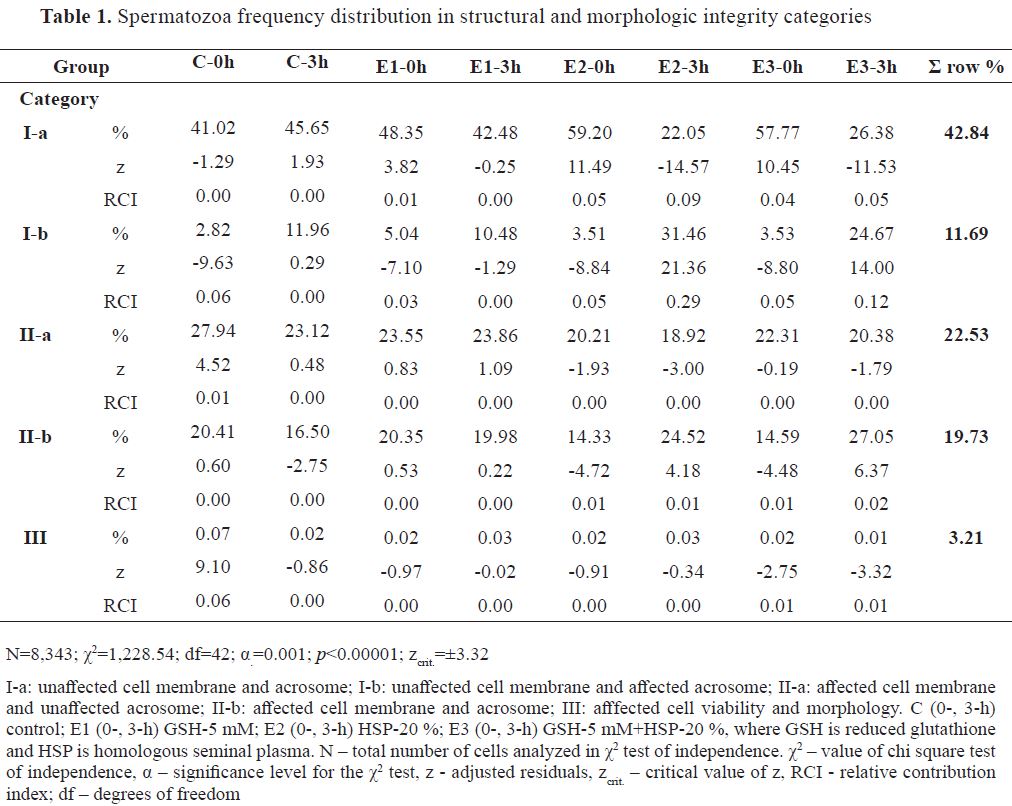
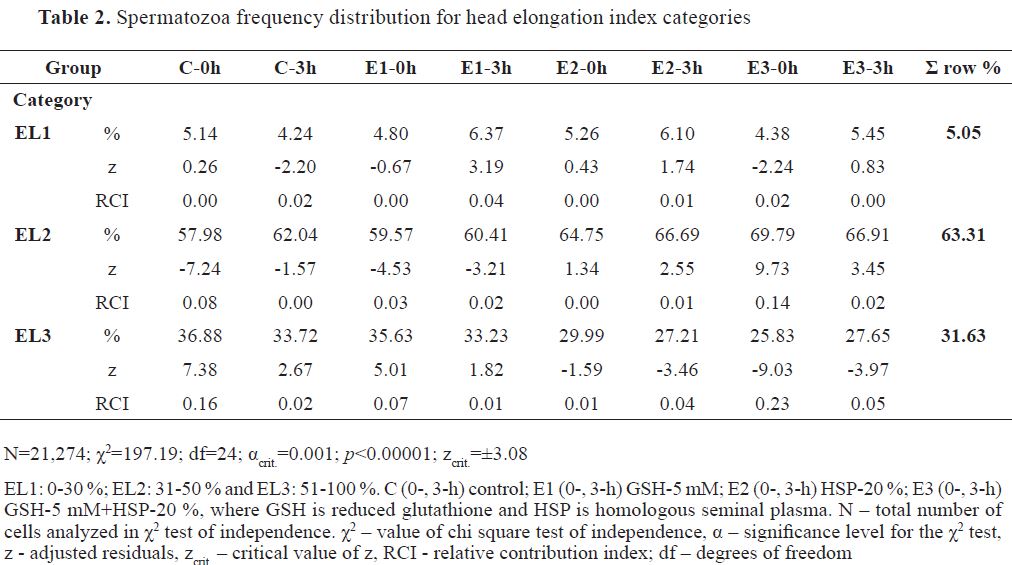
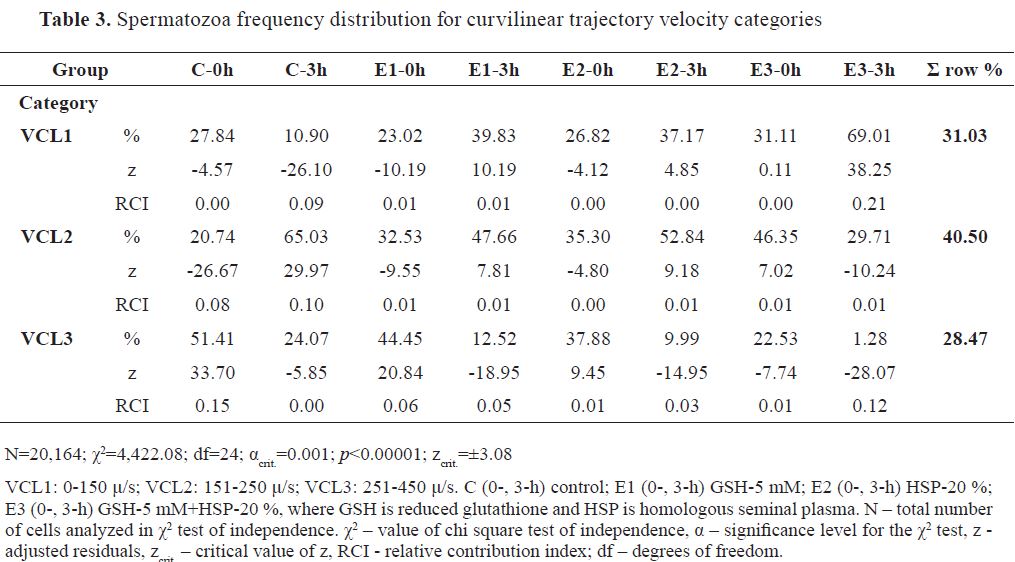
The major percentage of analyzed spermatozoa for EL was distributed in EL2 (63.31 %), almost one- third in EL3 (31.63 %), and the smallest portion in the EL1 category (5.05 %). Frequency distribution of spermatozoa (N=21,274) is shown in
Table 2. E3-0h had highly positive z from the omnibus chi-square (χ2=197.19) for EL2 spermatozoa ( f=69.79 %, RCI=0.14) and highly negative for EL3 spermatozoa ( f=25.83 %, RCI=0.23). This was also evident in E3-3h, but with much smaller positive ( f=66.91 %, RCI=0.02) and negative z ( f=27.65 %, RCI=0.05) for the same spermatozoa EL categories. C-0h had the highest positive z from the omnibus chi-square for EL3 ( f=36.88 %, RCI=0.16) and negative for EL2 category ( f=57.98 %, RCI=0.08). E1-0h had a reflection in the same categories as the control, but with smaller positive ( f=35.63 %, RCI=0.07) and negative z ( f=59.57 %, RCI=0.03), respectively. Following 3 hours (E1-3h), the positive z was reflected on EL1 ( f=6.37 %, RCI=0.04), whereas the negative on EL2 spermatozoa ( f=60.41 %, RCI=0.02).
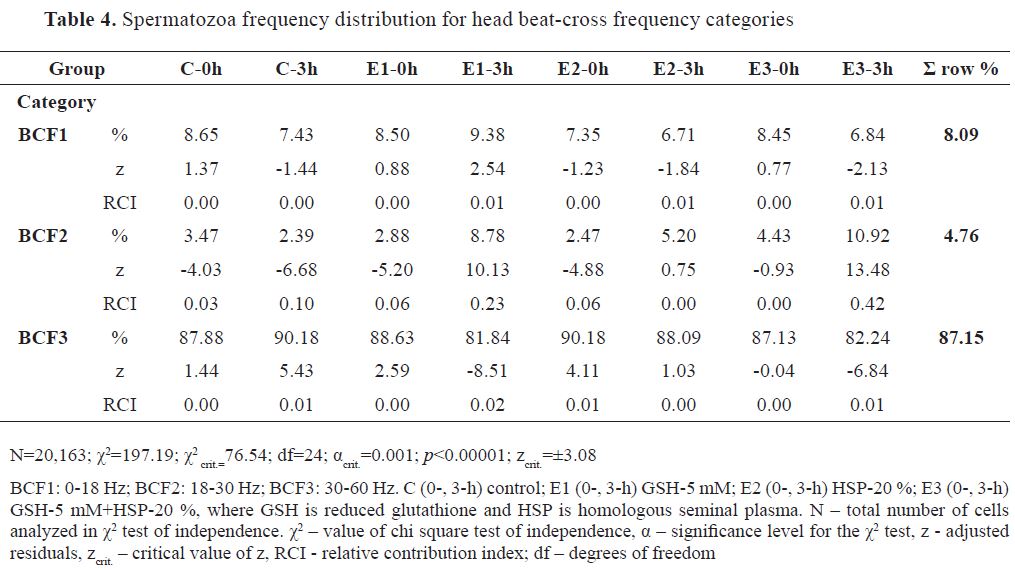
Spermatozoa velocity and tail beat-cross frequency indices
The distribution of spermatozoa in VCL categories from highest to lowest was in the following order: VCL2 (40.50 %), VCL1(31.03%), and VCL3 (28.47 %). The frequency distribution of analyzed cells (N=20,164) is presented in
Table 3 (χ2 =4,422.08).
E3-3h had the highest positive z and RCI for VCL1 ( f=69.01 %, RCI=0.21), followed by control extender group at 0 hours (C-0h) in VCL3 (f=51.41 %, RCI=0.15) and at 3 hours (C-3h) in VCL2 ( f=65.03 %, RCI=0.10). Significantly positive z values with lower RCIs (≤ 0.06) were observed for E1-0h ( f=44.45 %, RCI=0.06) and E2-0h ( f=37.88 %, RCI=0.01) in VCL3, for E1-3h ( f=47.66 %, RCI=0.01), E2-3h ( f=52.84 %, RCI=0.01) and E3-0h ( f=46.35 %, RCI=0.01) in VCL2, and for E1-3h ( f=39.83 %, RCI=0.01) and E2-3h ( f=37.17 %, RCI < 0.01) in VCL1. The highest negative z with highest RCI was detected for E3-3h in VCL3 ( f=1.28 %, RCI=0.12). Other negative z values with lower RCIs wereobservedforC-3h( f=10.90 %,RCI=0.09),E1-0h ( f=23.02 %, RCI=0.01), C-0h ( f=27.84 %, RCI< 0.01), and E2-0h ( f=26.82 %, RCI< 0.01) in VCL1, for C-0h ( f=20.74 %, RCI=0.08), E1-0h ( f=32.53 %, RCI=0.01), E3-3h ( f=29.71 %, RCI=0.01) and E2-0h ( f=35.30 %, RCI<0.01) in VCL2, and for E1-3h ( f=12.52 %, RCI=0.05), E2-3h ( f=9.99 %, RCI=0.03), E3-0h ( f=22.53 %, RCI=0.01) and C-3h ( f=24.07 %, RCI<0.01) in VCL3.
Spermatozoa distribution in BCF categories was skewed towards the highest value range category (BCF3, 87.15 %, BCF1, 8.09 %, and BCF2, 4.76 %). Frequency distribution of analyzed cells (N=20,163) is presented in
Table 4 (χ2 =371.42). In BCF1, none of the groups has had significant ±z. The dominant RCI for positive z values was observed for E3-3h ( f=10.92 %, RCI=0.42) and E1-3h ( f=8.78 %, RCI=0.23) in BCF2. Lower RCI for positive z was also observed for C-3h and E2-0h in BCF3, having identical values ( f=90.18 %, RCI=0.01). Dominant RCI for negative z was observed for C-3h ( f=2.39 %, RCI=0.10), followed by E2-0h ( f=2.88 %, RCI=0.06), E2-0h ( f=2.47 %, RCI=0.06) and C-0h ( f=3.47 %, RCI=0.03) in BCF2 category. In BCF3, significant RCI for negative z was observed for E1-3h ( f=81.84 %, RCI=0.02) and E3-3h ( f=82.24 %, RCI=0.01).
Spermatozoa trajectory pattern indices
Spermatozoa distribution in ALH categories was in the following highest-to-lowest percentile order: ALH2 (58.94 %), ALH1 (29.89 %), and ALH3 (11.17 %). Frequency distribution of analyzed spermatozoa (N=14,315) is shown in
Table 5 (χ2=611.02).
The highest RCI for positive z was found for C-0h ( f=17.09 %, RCI=0.13) in ALH3, and for E3-0h ( f=39.23 %, RCI=0.10) and E3-3h ( f=43.65 %, RCI=0.10) in ALH1. Smaller RCIs were detected for E1-3h ( f=16.64 %, RCI=0.07) and C-3h ( f=13.45 %, RCI=0.02) in ALH3, for C-0h ( f=63.13 %, RCI=0.01) and C-3h ( f=63.45 %, RCI=0.01) in ALH2, and for E2-0h ( f=34.45 %, RCI=0.02) in ALH1.
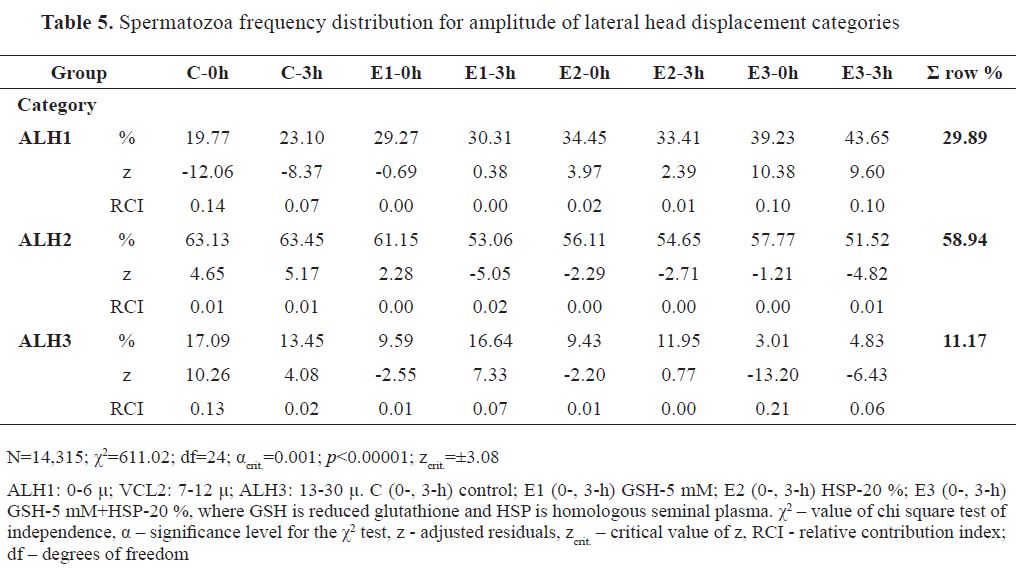
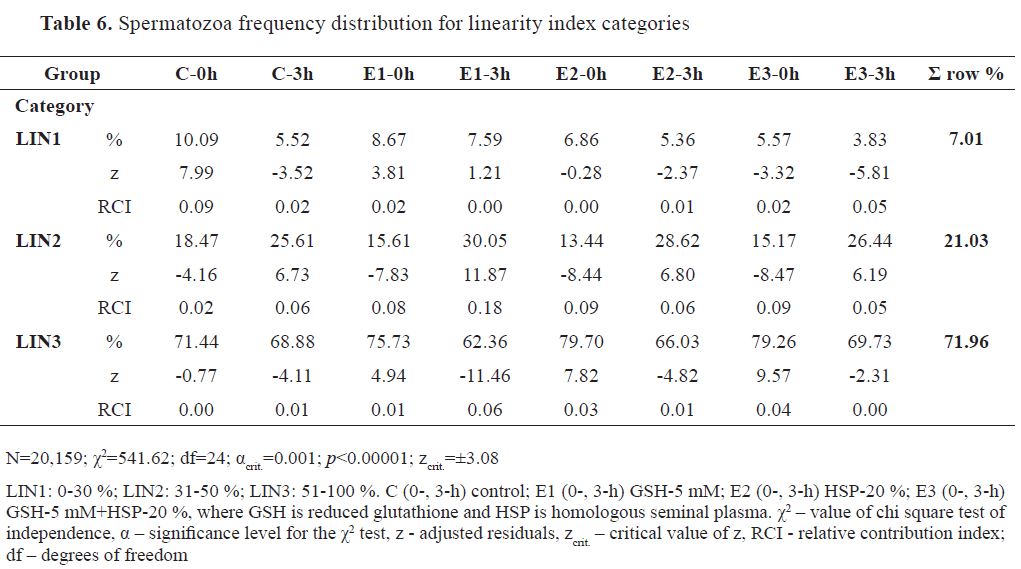
Spermatozoa percentile distribution among linearity categories were in the following highest-to- lowest order: LIN3 (71.96 %), LIN2 (21.03 %), and LIN1 (7.01 %). Frequency distribution of analyzed spermatozoa among groups (N=20,159) is shown in
Table 6 (χ2=541.61). The highest RCI for positive z was for E1-3h ( f=30.05 %, RCI=0.18) in LIN 2, followed by C-0h in LIN1 ( f=10.09 %, RCI=0.09). E1-0h ( f=8.67 %, RCI=0.02) in LIN1, E3-3h ( f=26.44 %, RCI=0.05), E2-3h ( f=28.62 %, RCI=0.06), and C3-3h ( f=25.61 %, RCI=0.06) in LIN2, E3-0h ( f=79.26 %, RCI=0.04), E2-0h ( f=79.70 %, RCI=0.03) and E1-0h ( f=75.73 %, RCI=0.01) in LIN3 had positive z with RCIs ≤0.06. The most dominant RCI in negative z was detected in E2-0h ( f=13.44 %, RCI=0.09), E3-0h ( f=15.17 %, RCI=0.09) and E1-0h ( f=15.61 %, RCI=0.08) in LIN2. Other RCIs (≤0.06) were observed in E3-3h ( f=3.83 %, RCI=0.05), E3-0h ( f=5.57 %, RCI=0.02) and C-3h ( f=5.52 %, RCI=0.02) in LIN1, C-0h ( f=18.47 %, RCI=0.02) in LIN2, and E1-3h ( f=62.36 %, RCI=0.06), E2-3h ( f=66.03 %, RCI=0.01), C-3h ( f=68.88 %, RCI=0.01) in LIN3.
DISCUSSION
This research tested the effects of GSH-5 mM (E1), HSP-20 % (E2) and GSH-5 mM + HSP-20 % (E3) supplemented CP media in achieving HEF of thawed spermatozoa categories with intact morphology and retained pre-capacitating motility, and to identify possible effects of incubation time in the previous context. Samples were extended prior cryo-preservation with commercial custom- produced CP medium (animal-protein- and GSH- free). Following thawing, they were incubated at 37 °C and sampled in ten replicates at 0- and 3-hours incubation time (N=80).
Ejaculate handling during laboratory analysis and preparation for cryopreservation (adding semen extenders) increases the ratio between the spermatozoa and seminal plasma components (
29). Diluting the fresh ejaculates with cryopreserving extenders decreases the concentration of SP and its components, along with its positive effects on spermatozoa structural integrity, motility and fertilizing ability (
29). The addition of SP to highly diluted spermatozoa has been demonstrated to improve spermatozoa viability (
30). In our research, the addition of HSP (E2 and E3 extenders) to thawed ram spermatozoa via cryopreserving media (20 %) resulted in an increased proportion of cells (HEF) with intact plasma and acrosome membranes (VIAB1), which complies with the previous report. The use of control extender has resulted in HEF of cells with affected structural integrity (VIAB2-5), which confirms the negative effect of dilution on thawed ram spermatozoa as described by Mann et al. (
31). Bergeron et al. (
32) have isolated two protein fraction of ram SP (RSVP-14 and RSVP-20), which were reported to have a stabilizing effect on spermatozoa membranes, preventing capacitation (
33,
34). We suggested that the HEF of viable cells in E2 and E3 groups was due to these components of the HSP. Furthermore, E1 extender had a similar effect on sperm viability as the control, implying that the GSH component had an inconclusive role on thawed spermatozoa.
Sperm head elongation has been correlated with spermatozoa linearity, velocity and fertilizing capacity (
3,
35). It is known that the cold-stress of spermatozoa causes structural and morphometric changes of its heads and can, therefore, affect the overall fertilizing capacity of thawed samples (
36). In the context of the previous effect of HSP on the structural integrity of spermatozoa, the HEF in EL2, and LEF in EL3 for E3 extender indicated that the combined presence of HSP and GSH in the cryopreserving extender significantly decreased the spermatozoa frequency with lower elongation of the head. This effect was observed at both 0- and 3-hours incubation. An opposite effect was observed for C and E1 extenders, having HEF in EL3 and LEF in the EL2 category. Despite the negative effect of GSH in E1 extender, and the absence of significant effect with E2 extender, it was acknowledged that the combined presence of these components in the E3 extender was essential for the manifestation of the positive effect on sperm head elongation.
Spermatozoa motility pattern is used as a predictor for fertilizing ability (
37). In-vivo studies have confirmed that progressive movement or higher trajectory linearity and higher velocity are positively correlated to fertility and oocyte penetrating ability of spermatozoa (37). Motility, in general, is considered as one of the key predictors of male fertility (
4). The hyperactivated state of spermatozoa has been kinematically characterized by high values of VCL, ALH and low values of LIN (
27). Conversely, higher values of LIN and lower of VCL and ALH are correlated with higher fecundity of spermatozoa (
27). In the context of these statements, E3 extender at 0- and 3-hour incubation showed the highest positive effect on the motility pattern of thawed ram spermatozoa. In compliance with the criteria for the aforementioned CASA parameters (
27), the motility pattern of spermatozoa in E3 group showed the highest linearity of the trajectory. The control extender at 0-hours incubation had the highest negative effect on sperm trajectory pattern due to the high values of VCL and ALH, and low values of LIN for the majority of the sperm population. In combination with high values of BCF, these sperm cells were considered as hyperactivated and with no fertile ability. Due to the subjectivity of the individual CASA parameters, conclusive responses were interpreted only for these groups, which had an integral effect on all parameters.
Generally, the incubation time had a highly negative effect on structural integrity (VIAB) in all groups. Head elongation and motility patterns were considered to be positively affected by incubation time. We acknowledged that immediately following thawing, a significant number of cells underwent capacitation and hyperactivation which was reflected in high variability of the frequency distribution in the CASA motility parameters. This, in turn, resulted in interpreting the motility pattern as highly deviated for almost all groups a 0-hour incubation, except E3-0h. With incubation time the number of hyperactivated spermatozoa was decreased, hence distribution frequency of the remaining motile cells was less variable in the CASA parameter categories. Therefore, following 3-hours of incubation, spermatozoa had a more stable trajectory pattern which was considered as linear and progressive.
CONCLUSION
In conclusion, E3 extender added immediately after thawing at 0-hour incubation yielded a significantly high number of thawed ram spermatozoa with intact viability, morphology and unaffected motility pattern. Conversely, the control extender added after thawing at 0-hour incubation yielded a significantly high number of thawed ram spermatozoa with affected viability, morphology and hyperactivated motility. E2 extender at 0-hour incubation yielded a significantly high number of viable spermatozoa with affected motility patterns. At 3-hour incubation, all extenders yielded a similar number of thawed ram spermatozoa with unaffected motility patterns, but altered viability.
CONFLICT OF INTEREST
The authors declared that they have no potential conflict of interest with respect to the authorship and/or publication of this article.
ACKNOWLEDGEMENTS
The authors would like to acknowledge and express their gratitude to IMV technologies for their support in participating to this publication.