Apart from currently available therapeutics for the treatment of diabetes mellitus, much attention has been paid to discover phytochemicals from natural resources, mainly due to their low side-effects. Hypericum perforatum hairy root (HR) transformed with Agrobacterium rhizogenes A4 represent prospective experimental system enriched in xanthones, known as potent antidiabetic agents. Thus, the aim of this study was to evaluate HR extracts for their potential antihyperglycemic activity in streptozotocin (STZ)-induced diabetic rats, also compared to the effects of wild-growing Hyperici herba (HH). We conducted an acute-toxicity study, multiple dose study, and 24h blood glucose measurements after a single dose administration of HH and HR (200 mg/kg) in diabetic rats. Furthermore, we examined the effects of 14-days administration of HH and HR extracts on blood glucose levels, metabolic parameters, enzyme, and lipid status in healthy and diabetic rats. Both extracts produced a fall of about 70% in blood glucose level after 24h of administration. Two-week treatment with HH and HR induced a significant decrease (70-72%) in blood glucose levels. Moreover, we found an improvement of the dysregulated metabolic parameters (body weight, food, and water consumption and urine output). Serum enzyme (AST, ALT, and γ-GT) and lipid profile parameters (CHOL, TAG, and HDL) were also improved by both extracts. These findings might provide a new insight for managing diabetic hyperglycemia and dysregulated serum enzyme and lipid profile, using extracts from transgenic roots cultures from H. perforatum.
Although different hypoglycemic drugs have been synthesized for the treatment of diabetes mellitus, many synthetic drugs have a number of serious side effects (
1). The management of hyperglycemia with minimal side effects and low costs is still a challenge to the medical system (
2). Comparatively low side-effects and low-cost phytochemicals from natural resources open new avenues for the treatment of various diseases including diabetes (
3).
Hypericum perforatum L. represents one of the best studied medicinal plants throughout the world with well-characterized bioactive metabolites and pharmacological properties (
4, 5). Consequently,
H. perforatum based products are prepared from Hyperici herba (HH) crude material as a source of natural bioactive pharmaceuticals. The
H. perforatum extracts contain naphthodianthrones, flavonol glycosides, biflavonoids, phloroglucinol derivatives, and xanthones (
4) that are responsible for antidepressant, anti-inflammatory, antiviral, anticancer, antibacterial, and antidiabetic activities (
6). Among these bioactive compounds, xanthones are known as the most potent antidiabetic agents (
7, 8, 9, 10, 11).
In the last decade,
H. perforatum extracts were found to have antihyperglycemic and antidiabetic activity in different animal models of diabetes (
12, 13, 14, 15). Still, the contribution of xanthones to the antidiabetic activity of
H. perforatum has not been considered due tо the apprehension that this class of compounds is mainly accumulated in the roots and only trace amounts have been found in the field-grown plants and commercially available products (
16).
In the search of alternatives for sustainable production of bioactive compounds,
Agrobacterium rhizogenes - mediated transformation of
H. perforatum represents an effective biotechnological approach for increasing production of existing secondary metabolites, as well as for biosynthesis of new bioactive components (
17). In that sense, genetic transformation of
H. perforatum with
Agrobacterium rhizogenes (A4 and A4M70GUS) leads to the establishment of
H. perforatum hairy root (HR) cultures that accumulated significant quantities of various xanthones (
18, 19).
Even though
in vitro antioxidant and radicalscavenging properties of
H. perforatum HR extracts have already been reported (
17), the antidiabetic activity of those cultures has never been explored. Thus, the abundance of various xanthones in
H. perforatum HR cultures could make them potential candidates for evaluation of their yet unknown
in vivo antidiabetic property in streptozotocin (STZ)-induced diabetic rats.
To the best knowledge of the authors, this was the first conducted study of the antihyperglycemic properties of xanthone-rich HR extracts. The aim of the study was to compare the effects of HH and the xanthone-rich HR extract by conveying basic toxicity test, blood glucose regulation assessment during single and multiple dose treatments, basic metabolic parameters regulation assessment (body weight, food consumption, water uptake, and urine output), hepatic damage key enzyme markers assessment (AST, ALT and γ-GT), and serum lipid profile assessment (CHOL, TAG and HDL).
MATERIAL AND METHODS
Plant materialSeeds from
H. perforatum wild-growing plants (voucher number 060231), collected from a natural population in the Republic of North Macedonia, were used for the establishment of in vitro grown seedlings. The experimental protocols for
Agrobacterium rhizogenes A4 transformation of
H. perforatum and induction of HR cultures were described in the study of Tusevski et al. (18).
Preparation of extractsOne-month-old
H. perforatum HR (cultured in liquid MS/B5 medium) were collected and lyophilized to absolutely dried state. The extraction of phenolic compounds from lyophilized plant material (0.2 g) was performed in the presence of 80% (v/v) CH3OH by using ultrasonic bath for 15 minutes (20). The methanolic extracts were then centrifuged at 15000 g for 15 minutes, and the resulting supernatants were evaporated to dryness under vacuum (50 mbar) at room temperature using rotary evaporator system. The dried extracts were dissolved in 0.3% carboxymethylcellulose-CMC (w/v in distilled water) at a concentration of 20 mg/mL. The identification and quantification of phenolic compounds in HR and HH methanolic extracts was previously performed using highperformance liquid chromatography (HPLC), coupled with diode-array detection (DAD) and tandem mass spectrometry (MSn) with electrospray ionization (
18).
AnimalsHealthy adult Wistar rats (weighted 200-250 g, aged 14-16 weeks) obtained from the Vivarium of the Faculty of Natural Sciences and Mathematics, Skopje, were kept under standard environmental conditions. All experiments were performed in accordance with the current ethical norms approved by the Animal Ethics Committee of the University “Ss. Cyril and Methodius”, Skopje, R. North Macedonia (03-2323/2), following the International Guiding Principles for Biomedical Research Involving Animals, as issued by the Council for the International Organizations of Medical Sciences. The anesthetic-sodium thiopental (45 mg/kg body weight) was applied according to the standards given by the guide of the EC Directive 86/609/EEC and 2010/63/EU.
Acute toxicity studyThe acute oral toxicity study was performed on healthy Wistar rats of both sexes, according to the Organization for Economic Co-operation and Development (OECD) Guideline no. 423. Group 1 (control group, n=6) received only vehicle, while Group 2 (n=6) received multiple doses of HR extract administration (via feeding needle), the animals were observed for three hours for any changes in the autonomics, general behavioral, and neurological profiles. The observations were continued for the subsequent 24 h in three-hour intervals for mortality, and daily for 14 days.
Experimental designExperimental diabetes was induced by single intraperitoneal injection freshly dissolved STZ, (Sigma Aldrich, Bangalore) (45 mg/kg) in cold citrate buffer (pH 4.5, 0.1 M). Diabetic rats were confirmed three days after the STZ injection. After seven days of STZ administration, rats with fasting blood glucose above 15 mmol/L were considered diabetic and were included in the study. The hypoglycemic drug glibenclamide (Glb) (2.5 mg/kg) was used as a positive control during the study. All treatments were administered through oral route via feeding needle.
Dose-dependent studyDose dependent study was performed to evaluate the effects of different doses of the HH and HR extract on blood glucose levels in diabetic rats. The diabetic rats were randomly divided into seven groups (n=6), fasted overnight, and administered 0.3% CMC (D), HH (DH), and HR (DHR) extracts (100, 200, and 300 mg/kg). Blood glucose level was measured using glucometer in blood samples collected from tail prior to extract administration as well as 8 hours after.
Single-dose studyIn this experiment, the regulation of glycemia with single application of HH and HR extracts in diabetic rats was monitored. The rats were randomly assigned into 5 groups of six animals each. I. Normal control rats (C) – placebo treated with water. II. Diabetic control rats (D) - placebo treated with 0.3% CMC. III. Diabetic rats treated with Glb (DGlb) IV. Diabetic rats treated with
H. perforatum extract (DH) (200 mg/kg body weight); V. Diabetic rats treated with hairy roots extract (DHR) (200 mg/kg body weight). All the rats were fasted for 8 h prior to the treatment, but were allowed a free access to water before and throughout the experiment. Blood glucose level was measured using glucometer in blood samples collected from tail vein prior to the extract administration (0 h) as well as 1, 2, 4, 6, 8, 12, and 24 h after.
Multiple-dose studyThis experimental design was employed to investigate the effect of multiple-dose treatment (14-day treatment) with HH and HR extracts in healthy and diabetic rats, comprising eight rats in each group:
C - Normal control, treated with water.
CH - Healthy rats treated with HH extract (200 mg/kg body weight)
CHR - Healthy rats treated with HR extract (200 mg/kg body weight)
D - Diabetic control treated with 0.3% CMC
DGlb - Diabetic rats treated with Glb (2.5 mg/kg body weight)
DH - Diabetic rats treated with HH extract (200 mg/kg body weight)
DHR- Diabetic rats treated with HR extract (200 mg/kg body weight).
All treatments were administered once daily, in 8 h fasted rats (water
ad libitum) which were then allowed a free access to water and food after the treatment. Fasting blood glucose (before the treatment) was measured using glucometer in blood samples collected from tail vein prior to the extract administration during the 14-day treatment.
The body weight changes and food consumption were measured during the experiment at day 1, 7 and 14. Urine output collections as well as water and food consumptions were evaluated using metabolic cages (Ugobasile, 41700, Verase, Italy) at the last day of the experiment.
Blood was collected from the dorsal vein in serum and plasma separation tubes.
Biochemical analysisGlycemic profile was estimated trough quantification of glucose levels using GOD method, colorimetric kit (Human, Germany). Also, serum level of triglycerides (TAG), total cholesterol (TC) and high-density lipoprotein (HDL), serum glutamicoxaloacetic transaminase (SGOT/AST), serum glutamicpyruvic transaminase (SGPT/ALT) and γ-glutamyltransferase (γ –GT) were evaluated using commercially enzymatic tests (Human, Germany).
Statistical analysisAll values were expressed as mean ± SEM or SD. Statistical analysis was performed by one-way analysis of variance (ANOVA) followed by Tukey’s post hoc test. The results were considered statistically significant at p<0.05. GraphPad Prism (version 9) software was used for statistical analysis.
RESULTS
Acute toxicity studyHR-treated animals of both sexes did not show any alterations in their neurobehavioral patterns, health status, or mortality at the highest used dose of 2000 mg/kg. When compared to vehicle-treated group, there were no significant changes in the body weight and food intake. Hence, the extract was suggested to be safe for consumption even at the dosage of 2000 mg/kg.
Dose dependent-studyFollowing the results of the acute toxicity test, different doses of both extracts (100 mg/kg, 200 mg/kg and 300 mg/kg) were used to determine their effectiveness (
Table 1). The diabetic group showed non-significant reduction in blood glucose level after eight hours. A single treatment with HH extract (applied in three doses) led to a significant decrease in blood glucose concentration after eight hours of application (-22.7%, -29.4% and -26.9%, respectively). Similarly, treatment with three doses of HR extract resulted in a significant reduction in blood glucose concentration of -28.6%, -31.6% and -21.7%, respectively. However, with this experiment we found that the dry extract from HR shows reduced solubility in 0.3% CMC at a dose of 300 mg/kg. Accordingly, in the subsequent experiments, a dose of 200 mg/kg was used for both extracts.
Single dose-studyFigure 1 shows the effects of single dose treatment with HH and HR extracts in STZ-diabetic rats. The blood glucose level of the healthy control animals was significantly reduced by about 14.6%. On the other hand, diabetic animals showed high glycemia in all measurements made in the first 12 hours (above 15 mmol/L) which in the last measurement (24 hours) showed a decrease to 9 mmol/L (D0:D24, -53.3%, p<0.05). The three treatments of diabetic animals (DGlb, DH and DHR) showed similar dynamics. The first decrease in the glycemia was observed after the first hour of treatment and already in the eighth hour of treatment the glucose concentration was reduced to normal values (about 7 mmol/L). This normoglycemia was maintained until the 24th hour. Namely, at 24 hours, the measured glycemia in Glb, HH, and HR-treated animals was significantly reduced by -68.9%, -69.5% and -69.9%, respectively, compared to the glycemia measured before the beginning of treatment (0 h).
Figure 1. Changes in blood glucose levels in diabetic rats treated with single dose of Glb, HH and HR extract during 24-hour period. Data are presented as the mean ±SD. *Significant difference (p<0.05) compared to glucose measured in 0 hours
Multiple-dose studyFigure 2 shows the effect of the two-week treatment with HH and HR extracts on the blood glucose level in healthy and STZ-diabetic rats. All control groups (C, CH, and CHR) were normoglycemic (approximately 6.77 mmol/L) throughout the experiment (C0:C14; CH0:CH14; CHR0:CHR14, n.s.).
Figure 2. Changes in fasting blood glucose during two-week treatment of healthy and diabetic rats with HH and HR extracts. Data are presented as the mean ±SD. *Significant difference (p<0.05) compared to blood glucose concentration measured on day 0 of the treatment
The diabetic control group demonstrated hyperglycemia (glycemic values greater than 20 mmol/L) during the two-week period, while the 14-day treatment of diabetic animals with Glb caused a decrease in glycemia of 31.1 %, which is statistically insignificant. The treatment of diabetic animals with HR extract caused a significant reduction in glycemia of about 33.5% and 35.1% on the 2nd and 4th day of treatment, respectively. Both HH and HR treatments caused a significant reduction in glycemia in diabetic animals for about 43% and 50% (for DH and DHR, respectively) on the 6th day of treatment. At the end of the experiment (day 14), HH extract caused a decrease in glycemia by 70.3% (p<0.05), while treatment with HR extract by 71.8% (p<0.05).
The plasma glucose in healthy and STZ-diabetic rats treated with HH and HR extracts measured at the end of the 14-day treatment are presented on
Fig. 3. Both treatments of control animals did not lead to significant changes in blood glucose concentration. The treatment of diabetic animals with Glbsignificantly reduced glucose concentration by -38.5% (D:DGlb, p<0.05), but still did not lead to normoglycemia (C:DGlb p<0.05). The treatments of diabetic animals with HH and HR extracts reduced glucose concentrations by 70.1% and 72.7%, respectively (D:DH, D:DHR, p <0.05), achieving normoglycaemia (C:DH, C:DHR, ns).
Figure 3. Changes in plasma glucose concentration in healthy and diabetic rats with HH and HR extracts. Data are presented as the mean ±SD. *Significant differences (p<0.05): a-compared to C; b-compared to D; c-compared to DH
The effect of the two-week treatment of HH and HR extracts on the body weight (A) and food consumption (B) in healthy and STZ-diabetic rats are shown in
Fig. 4. Namely, during the 14-day treatment, in all groups of control animals (C, CH, and CHR) there was an average increase in body weight of about 15%, p<0.05. The diabetic controls showed a decrease in body weight of -29.1% (D1: D14, p<0.05), while treatment with Glb caused only slight improvement and less pronounced changes in body weight of -13.5% (p<0.05). The HH and HR treated diabetic animals showed no significant (0.2% and 0.8%, respectively) changes in body weight between the first and 14th day of the experiment. The food consumption was increased by 45.5-57.3% in control animals (C, CH, CHR) while in the diabetic group the food consumption was 1.35 times higher than at the beginning of the treatment (p<0.05). The Glb-treated group showed a 78.3% increase in food intake (p<0.05). In diabetic animals treated with HH and HR, the food consumption was lower than the consumption in diabetic controls and those treated with Glb. Namely, the groups treated with HH and HR extracts had significant increase of 45.1% and 37.7% respectively.
Figure 4. Changes in body weight (A) and food consumption (B) measured on 1st , 7th and 14th day of the treatment in healthy and diabetic rats with HH and HR extracts. Data are presented as the mean ±SD. *Significant difference (p<0.05) compared to day 1 of the treatment
The effect of the two-week treatment with HH and HR extracts on water consumption (A) and urine output (B) in healthy and STZ-diabetic rats is presented on
Fig. 5. No significant change in water consumption and urine output was observed in control animals treated with HH and HR extract (C:CH, C:CHR, ns). On the other hand, experimental diabetes led to a two-fold increase in water consumption and 3.75-fold increase in urine output (p<0.05). The treatment with Glb did not lead to changes in water consumption (D:DGlb, n.s.), but caused a significant reduction in urine output by 33.6% compared with diabetic controls (D:DGlb, p<0.05). The HH and HR-treatments of diabetic animals caused a significant reduction in water consumption (by 61.6% and 59.3%, respectively) and urine output (by 51% and 76.3%, respectively). However, the treatment with HR was more efficient in urine output reduction (DH:DHR, p<0.05).
Figure 5. Changes in water consumption (A) and urine output (B) in healthy and diabetic rats treated with HH and HR extracts. Data are presented as the mean ±SD. *Significant differences (p<0.05): a-compared to C; b- compared to D; c-compared to DH
The effect of HH and HR extracts on the serum lipid profiles in STZ-induced diabetic rats is presented in
Table 2. Compared with the normal control group, there was a significant (p<0.05) increase in the CHOL and TAG concentration, in the diabetic control rats, but no significant changes were found in HDL concentration. The treatment of diabetic animals with Glb caused a decrease in serum CHOL (D: DGlb, -25.7%, p<0.05) and serum TAG (D: DGlb, -31%, p<0.05), but had no significant effect on HDL concentration (D: DGlb, ns). The treatments of diabetic animals with HH and HR led to a significant reduction in cholesterol concentration (D: DH, -29.6% p<0.05; D: DHR, -36.9%, p<0.05) and TAG (D: DH, -47.8%, p<0.05; D: DHR, -41.5%, p<0.05) but showed no significant effect on HDL concentration.
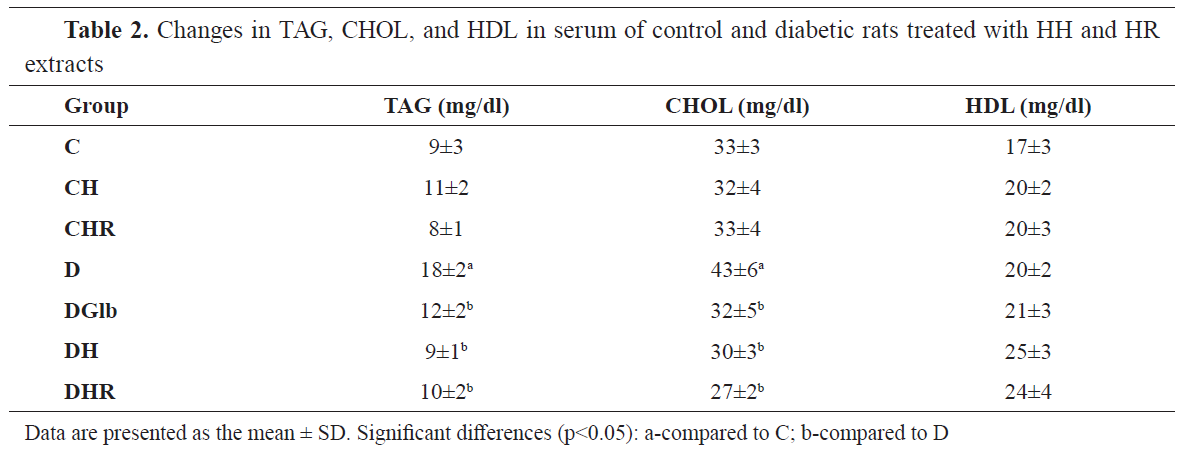
The effect of HH and HR extracts on the serum enzyme profile in the STZ-treated diabetic rats is presented in
Table 3. Treatments of healthy animals with HH and HR extracts did not cause significant changes in AST and ALT activity. The treatment of healthy animals with HH caused a significant increase in γ-GT enzyme activity (C: DH, 20.8%, p<0.05), whereas treatment with HR had no significant effect on this parameter. There was a significant increase in the activity of ALT (C: D, 63.4%, p<0.05) and γ-GT (C: D, 64.2%, p<0.05), but did not cause any significant changes in AST activity (C: D, ns) in the diabetic control group. The Glb-treatment resulted with non-significant treatments of diabetic animals with HH and HR resulted in a significant reduction in ALT activity (D: DH, -25.9% p<0.05; D: DHR, -23.1%, p<0.05) and γ-GT (D: DH, -14.6% p<0.05; D: DHR, -21.2%, p<0.05), but did not cause significant changes in AST.
DISCUSSION
The present study highlighted the hypoglycemic action of the Hairy roots extract in diabetic-induced rats. The single-dose application of HH, HR reduced the blood glucose levels of diabetic rats by about 70% after 24 h. With this reduction, the treatments lead to a state of normoglycemia in the treateddiabetic animals. These effects were comparable with those of Glb, oral hypoglycemic agent of the sulphonylurea group widely used in the treatment of diabetes mellitus (
21). Similarly, in the multiple dose study, the difference between the initial and final fasting glucose of HH and HR treated diabetic groups revealed a significant reduction in blood glucose. At the end of the experiment (day 14), HH extract caused a decrease in glycemia by 70.3% (p<0.05), while treatment with HR extract by 71.8% (p<0.05). In this experiment, the effects of both HH and HR treatments were more emphasized than those of the referent hypoglycemic agent glibenclamide used in this study.
The glucose-lowering properties of
H. perforatum extracts аre already well known (
13, 14, 15) but these are the first findings about the hypoglycemic effect of HR extract in diabetic rats.
H. perforatum hairy roots are unique model for production of xanthones and previous HPLC analyses clearly demonstrated that γ-mangostin, mangiferin, and other prenylated and oxygenated xanthones in
H. perforatum HR cultures are common defense compounds in response to
Agrobacterium transformation (
17). Several studies of plant extracts rich with γ-mangostin and prenylated and oxygenated xanthones report strong hypoglycemic effects in STZ diabetic rats (
9, 10, 11). One of the many pharmacological effects of mangiferin is the reduction of plasma glucose in different animal models with diabetes (
7) via several unique mechanisms including inhibition of glucosidase (
22), which could prevent overloaded carbohydrates from being converted to glucose and absorbed in the intestine.
Some previous results also manifested that
H. perforatum wild-growing roots showed strong
in vitro inhibitory activity against carbohydrate hydrolyzing enzymes α-glycosidase and α-amylase and thus, could be used for reduction of postprandial hyperglycemia (
18). According to Fouotsa et al. (
23), the antihyperglycemic properties of xanthones from
H. perforatum could be related to the structural characteristics of xanthone nucleus (prenylation and hydroxylation pattern) that are essential for effective α-glycosidase and α-amylase inhibitory activity.
Streptozotocin - induced diabetes is characterized by hyperglycemia, severe changes in metabolic parameters like body weight loss, polydipsia, polyphagia, and polyuria (
24). In the present study, we found significantly reduced body weight and increased water and food consumption along with increased urine output in the diabetic rats compared to the non-diabetic rats, indicating that diabetes was effectively induced. In the Glbtreated diabetic group, although less emphasized, we found similar trend of changes as those found in the diabetic control group. Similar results for the effects of Glb on these metabolic parameters in STZ-diabetic rats have been reported by Moodley et al. (
25). Unlike the Glb treatment, both HH and HR extracts improved body weight, decreased food consumption and significantly reduced water intake and urine output in diabetic rats, which might result from improved glycemic control.
Abnormal lipid metabolism, leading to accumulation of plasma LDL, VLDL, and total cholesterol as well as decreased HDL-cholesterol, is commonly associated with diabetes mellitus (
26). In the STZ diabetic group we found an increase in the level of CHOL and TAG, but no significant changes in HDL concentration when compared to the normal control group (
Table 2). The treatments of diabetic animals with HH and HR led to a significant reduction in cholesterol and TAG, but did not alter the HDL concentration. It is known that
H. perforatum extract can modulate blood lipid abnormalities at the experimental level (
12, 13, 15). Several of the xanthones which are present in the HR extract can modulate the lipid metabolism (mangiferin, mangosteen, and xanthones with 1,3,5,6-tetrahydroxyoxanthantone structure) (
18, 19). Namely, mangiferin normalizes the levels of cholesterol, triglycerides, free fatty acids, improves the balance between LDL and HDL in diabetic animals (
27, 28, 29), and improves the lipid profile in humans (
30). Alpha mangosteen applied in nanoconcentrations for only one week causes a decrease in the concentration of cholesterol and LDL in diabetic mice (
31).
Finally, we estimated the considered serum enzyme profile like biomarkers of hepatocellular damage. Elevated levels of liver transaminases, alanine aminotransferase (ALT), aspartate aminotransferase (AST), and γ-glutamyl transferase (γ-GT) are associated with fatty liver disease and hyperglycemia in diabetes (
32, 33). The treatment of diabetic rats with both HH and HR significantly reduced ALT and γ-GT levels (
Table 3), suggesting that they can ameliorate STZ-induced hepatocyte injury in diabetic rats. Our results are consistent with those of Mohammad et al. (
15) which in addition to the effects on lipid metabolism have shown that
H. perforatum extracts normalize the activities of AST and ALT in diabetic animals. On the other hand, xanthones are known to possess a hepatoprotective effect on diabetic rats (
34, 35).
CONCLUSION
In conclusion,
Hypericum perforatum hairy roots extract exhibited potent antihyperglycemic effect in STZ-induced diabetic rats without inducing hypoglycemic state. HR extracts manifested regulation of metabolic parameters, as well as serum lipid and enzyme profile in multiple dose treatment of diabetic rats. These multiple advantageous properties of the HR extract could be of greater therapeutic benefit in the management of diabetes mellitus. Still, further studies are necessary to evaluate the effects of HR-extracts on insulin secretion and the carbohydrate metabolism.
CONFLICT OF INTEREST
The authors declare that they have no potential conflict of interest with respect to the authorship and/or publication of this article.
ACKNOWLEDGMENTS
This research was supported by the Institute of Biology, Faculty of Natural Sciences and Mathematics in Skopje.
AUTHORS’ CONTRIBUTION
ER, BM and SDK conceived and designed the study, supervised the experiments and data analysis, interpreted the results, performed a major part of the experiments and wrote the manuscript. OT and SGS prepared the plant extracts, participated in designing the study, and contributed to finalizing the manuscript.