The myocardium of diabetic subjects displays reduced HSP70 protein level and weak myocardial protection. However, the heart possesses an ability to produce heat shock proteins (HSPs) after exposure to sublethal heat stress. Acetylsalicylicacid (ASA) has the property of pharmacological induction of HSPs. We evaluated the common effects of single dose ASApretreatment, prior to heat preconditioning (HP), over carbohydrate metabolism-related enzymes and substrates in the heart of diabetic rats. Streptozotocin-diabetes caused significant decrease of HSP70 protein level, stimulation of the gluconeogenic processes and inhibition of glycolytic processes in the heart. HP-diabetic hearts have significantly higher HSP70 protein level, lower glycogen, glucose-6-phosphate content, glycogen phosphorylase and hexokinase activity, and higher glucose levels and PFK activity. ASA-pretreatment of HP-diabetic animals caused additional increase of HSP70, additional decrease of glycogen, glucose-6-phosphate, glycogen phosphorylase and hexokinase, and additional increase of glucose and PFK in the heart. In conclusion, HP is physiological inducer of HSP70 level in heart and tends to reverse carbohydrate - related disturbances in diabetic rats. ASA, given prior to HP, is a potent HSP70 co-inducer and causes additional increase of HSP70 protein level in heart. ASA, given in a combination to HP, have shown more evident protective effects against subsequent intense of stress.
Тhe heart and other tissues have an ability to produce heat stress proteins (HSPs) as a response to exposure to heat stress. These HSPs, especially HSP70 are responsible for adapting to stress and renders it more resistant to subsequent injury. Heat preconditioning is a powerful adaptive phenomenon, meaning that a sublethal stress (such as ischemia or heat stress) enhances its tolerance to a subsequent stress in the myocardium, which might be lethal otherwise (
1, 2). Heat preconditioning can restore the cellular homeostasis and may serve to protect the protein’s structure (
3, 4, 5), while the HSP70 synthesis is a essential part of the whole physiological response to the stressor (
6).
heat stress, a number of pharmacological treatments can induce HSP expression and thus prevent injury form extreme stress. In this sense, sodium salicylate, aspirin and indomethacin as a non-steroidal anti-inflammatory drug (NSAIDs), have been shown to modulate heat shock response by increasing the induction of HSPs (
7).
Aspirin (acetyl salicylic acid - ASA) is widely used for its broadly therapeutic antiinf lammatory and anti-pyretic properties. Moreover, sodium salicylate has the property of partially inducing the heat shock response in humans (
8). Namely, ASA up regulates expression of different HSPs family members in different species (
9). The modulation of the heat shock response by aspirin is related with the ability of this drug to potentiate the impact of hyperthermia and prolong thermotolerance (
10). It is important to stress that ASA-induced expression of HSP was been potentiated in a combination to heat stress. In this sense, ASA enhanced the induction of HSP70 in chicken myocardial cells exposed to heat stress, which was unable in the absence of heat stress (
11).
Very evident phenomenon in diabetes mellitus (DM) is the altered expression of molecular chaperones, since long-lasting hyperglycemia causes significant modifications of cellular proteins (
12). The increased sensitivity and vulnerability of the tissues in DM is due to the reduced response of HSPs (
13). According to Chen et al. (
14), diabetic myocardium is associated with reduced myocardial protection and reduced abundance of HSP70, while myocardial injuries, are exacerbated in diabetic patients.
Concerning ASA, it was found that highdose (100 mg/kg in drinking water) and longterm ASA (5 mounts) therapy of type-1 diabetic rats resulted in decrease in the glucose level, nonsignificant elevation in the serum insulin level and induced serum HSP70 (
15).
The aim of this study was to examine the effect of whole-body heat stress (physiological inductor) and acetylsalicylic acid treatment (pharmacological inductor) over HSP70 production in diabetic heart. Moreover, we hypothesized that induction of HSP70 can reduce or even normalize the carbohydrate metabolism -related disturbances in diabetic myocardium.
MATERIAL AND METHODS
Animals and tissue proceduresThis experimental work was performed with male Wistar rats (3-4 months old and body weight of 250–300 g, n=78). The animals were fed with standard laboratory chow and received water ad libitum. They were kept under a 12-hour light regime (6 a.m. - 6 p.m. light) during the whole experimental period. The animals were sacrificed always at the same time of the day (9-10 a.m.), with a standard laparothomic procedure, after induction of anesthesia with Na-thiopental narcosis (45 mg/kg), following the guidelines of the EC Directive 86/609/EEC. For the experimental purposes of this research, we isolated rat’s heart, washed with cold saline solution and immersed it in liquid nitrogen. We prepared tissue powder (at liquid N2-temperature) and the portions of it were kept at -80 °C. Before analyses, the tissue powder was homogenized with an ultrasonic homogenizer (Cole-Parmer Instrument- 4710) in a period of 7–10 s, always at 0–4 °C (in ice).
All experiments were performed in accordance with the current ethical norms approved by the Animal Ethics Committee of the Ss. Cyril and Methodius University in Skopje, North Macedonia (03-2323/3) following the Directive 2010/63/EU for the protection of animals used for scientific purposes of the European Commission.
Study design and treatmentsWe assessed the effect of ASA-pretreatment, followed by heat-preconditioning of healthy and diabetic rats. Thus:
Aspirin (Acetylsalicylic acid, ASA, Sigma) was given to the animals always 1 h before HS. Animals received 100 mg ASA/kg b.w, in a total 0.5 mL volume, administrated intraperitoneally, always freshly dissolved in water. For dissolving of ASA crystals, we added sodium carbonate until ASA had completely dissolved (the pH of the solution remained just below 7.0). The dosage and the application were selected according to Fawcett et al. (
7) and Locke and Atance (
16).
Heat stress (HS) or heat preconditioning (HP) were carried out by placing of the animals in special heat- chambers (45 min at 41±0.5 ºC). After the heat exposure, animals were left to recover 24 h at room temperature (20±2 ºC).
Single intraperitoneal injection of streptozotocin (STZ, 55 mg/kg body weight) was used for induction of experimental diabetes. STZ was freshly dissolved in 0.1 M citrate buffer, pH=4.5. Only animals with the clear diabetic symptoms (fasting glycemia levels higher than 15 mmol/L) 24-48 hours after the induction of the experimental diabetes were used in the experiment.
The diabetes was left to develop for different duration times - 2, 7 and 14 days, and this was defined by the following two criteria: 1. Minimum period for manifestation of the effect of administrated STZ (2 days after clear hyperglycemia) and optimal period for the development of diabetic complications (14 days); 2. Dynamics of induction of HSP after the heat stress: abundant HSP70 protein level in the first 24-48 h after the single HS, and further decrement 72 h after the HS (
6, 17).
Organization of the experimental groupsThe organization of the experimental groups according the treatments timing is shown in
Table 1. We used healthy animals and diabetic ones. Furthermore, healthy animals were subdivided into three subgroups: control animals (C), heatexposed control animals (HC) and ASA-pretreated heat-exposed control animals (AHC). Regardless of duration of diabetes (2, 7 or 14 days), diabetic animals were divided into nine subgroups: control diabetic groups (D2, D7 and D14); heatpreconditioned diabetic groups (HD2, HD7 and HD14) and ASA-treated heat-preconditioned diabetic groups (AHD2, AHD7 and AHD14). Each experimental group numbered 6-7 animals.
Analytical methodsHSP 70 protein levelThe protein level of HSP70 in heart tissue was determined with the use of quantitative immunoassay sandwich test (Enzo Life Science International, Inc.) in accordance with the manufacturer manual. Briefly, heart samples were homogenized with extraction buffer, with protease inhibitor (0.1 mM PMSF) added before. The homogenates were centrifuged at 21000 g/10 min/4 °C. The extracts were diluted, and both, extracts and standards were applied on microtiter plate wells, which were pre-coated with monoclonal antibody specific for inducible HSP70. HSP70 specific rabbit polyclonal antibody was used for detection of the captured HSP70, which were subsequently bounded by an anti-rabbit IgG secondary antibody. HSP70 was measured in a microplate reader (Bio- RadModel 680, Philadelphia, USA) at 450 nm and the results were presented as ng HSP70/mg protein. We also measured total proteins concentration in heart samples by Lowry method (
18).
Enzymes activities and substrates concentrationAll the enzymes activities and substrates concentration were measured in a homogenates of heart tissue powder, prepared in appropriate medium of homogenization. For determination of glucose, glycogen, and glucose-6-phosphate concentrations in the heart (
19) perchlorate homogenates were prepared and further neutralized with 5M K2CO3. We measured production of NADPH at 340 nm, in a reaction catalyzed by glucose-6-phoshate dehydrogenase. The activity of liver glycogen phosphorylase was as reported by Stalmans et al. (
20). The substrate mixture was prepared from 50 mM glucose-1-phosphate, 1% glycogen, 2.5 mM EDTA, 0.15M NaF and 0.5 mM caffeine (pH=6.0). For determination of hexokinase (HK) (
21) and phosphofructikunase (PFK) (
22) we measured the production of NADH at 340 nm. The amount of released inorganic phosphate was determined by Fiske and Subbarow (
23). The total quantity of the proteins was determined by Lowry et al. (
18) and bovine serum albumin was used as a standard. Enzyme activity was expressed as nmol Pi/min/mg prot. The substrate concentration was expressed as μmol/g tissue.
StatisticsThe results are presented as means ± SD. We used one-way ANOVA with Neuman–Keuls post hoc test to examine the statistical differences among the groups (Statistica 7 and Statgraph 3). In all tests, a probability level of p<0.050 was used as a significant difference.
RESULTS
HSP70 level in heartAs shown in
Fig. 1 and
Table 2, heat stress caused about 2.9-fold (188%) increase of HSP70 protein level in the heart (C: HC), and ASApretreatment of HS-animals induced about 6.8-fold (583%) increase of HSP70 (C: AHC). Experimental diabetes (both 2 days and 14 days) by itself, caused significant decrease of HSP70 protein level (for about 30-45%), but when diabetic animals were heat preconditioned, there was significant elevation of HSP70 (about 85%). Still, these changes are more evident by the common effects of ASA pretreatment and HP of diabetic animals (for 200-250%).
Figure 1. HSP 70 protein level in heart of heat-preconditioned intact and diabetic rats treated with acetylsalicyl acid. C - Control (intact) animals (n=6); HC - Control animals exposed to HS and recovered 24h at room temperature (n=6); AHC - Control animals treated with aspirin 1h before HS and recovered 24h at room temperature (n=6); D2, D7, D14 - Diabetic animals (n=6); HD2, HD7, HD14 - Diabetic animals exposed to HS and recovered 24h at room temperature before induction of diabetes (n=7 each group); AHD2, AHD7, AHD14 - diabetic animals treated with aspirin 1h before HS and recovered 24h at room temperature before induction of diabetes (n=7 each group).
Significant difference (p <0.050): a – relative to control animals (C:HC, C:D2, C:D7, C:D14); b - relative to diabetic animals (D2:HD2, D7:HD7, D14:HD14, respectively); c - relative to control animals exposed to HS (HC:AHC, HC:HD2, HC:HD7, HC:HD14); d - relative to diabetic animals exposed to HS (HD2:AHD2, HD7:AHD7, HD14:AHD14, respectively)
Glucose content, hexokinase activity and glucose-6-phosphate content in heartThere was a significant decrease of heart Glu content after exposure to HS (about 72%), but this decrease was less evident while in the HS-animals pretreated with ASA (about 57%) (
Fig. 2 and
Table 3). Experimental diabetes, in a function of duration (2, 7 or 14 days), caused a gradual decrease of heart Glu content (23%, 39% and 59%, respectively). Heat preconditioning of diabetic animals caused elevation of Glu content (27% in HD7 and 89% in HD14) compared to diabetic ones. This increase of heart glucose was more evident in ASA-treated HP-diabetic animals (52% in AHD7 and 116% in AHD14), with tendency to normalization to control values.
Figure 2. Glucose content (A), hexokinase activity (B) and glucose-6-phosphate content (C) in heart of heat preconditioned intact and diabetic rats treated with acetylsalicyl acid
Legend: As in Figure 1.
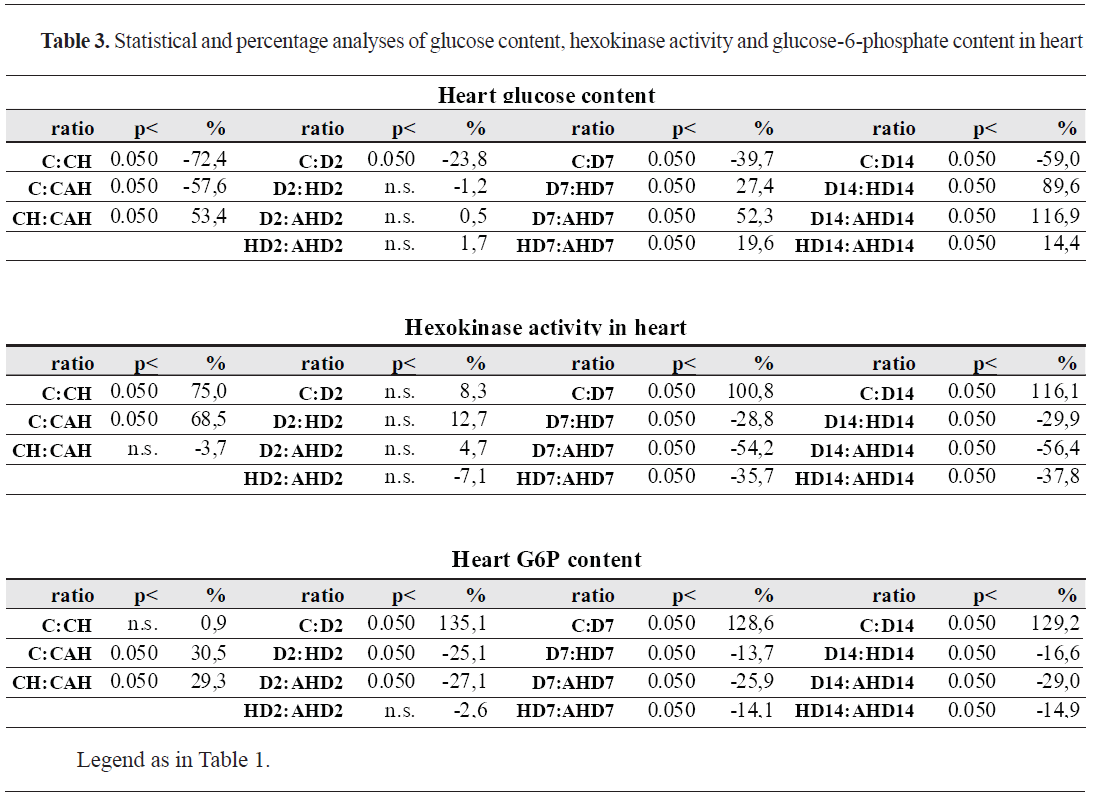
In contrast to the content of Glu in the heart, the activity of HK was significantly increased by HS (75%) and ASA treatment (68%) (
Fig. 2). Seven and 14-day diabetes caused significant increase of HK activity (100% and 116%, respectively). HP of diabetic rats resulted in decrease of HK-activity (for about 30%), which was more evident with ASAtreatment of HP-diabetic rats (about 55%), with almost tendency of normalization to control values.
HS did not caused any changes, but ASA treatment increased G6P content in control animals (
Fig. 2). Experimental diabetes (regardless of duration) caused an evident increase of G6P content (about 130%, regardless of duration of diabetes). HP of diabetic animals resulted in significantly lower G6P content (13-25%) and ASA treatment of HP-diabetic animals caused additional decrease of G6P content (25-29%).
Glycogen content, glycogen phosphorylase a and phosphofructokinase activity in heartAs shown in
Fig. 3 and
Table 4, HS caused a significant increase (69%) and ASA treatment of HS-animals resulted in additional increase of heart glycogen content (98%). Experimental diabetes (regardless of duration) caused an evident increase of heart glycogen content (about 6-fold). Heat preconditioning of diabetic animals resulted in significantly lower heart glycogen content compared to diabetic animals (abut 10-25%) and ASA treatment of HP-diabetic animals caused additional decrease of heart glycogen content (about 27-42%).
Figure 3. Glycogen content (A), glycogen phosphorylase a activity (B) and phosphofructokinase activity (C) in heart of heat-preconditioned intact and diabetic rats treated with acetylsalicyl acid
Legend: As in Figure 1.
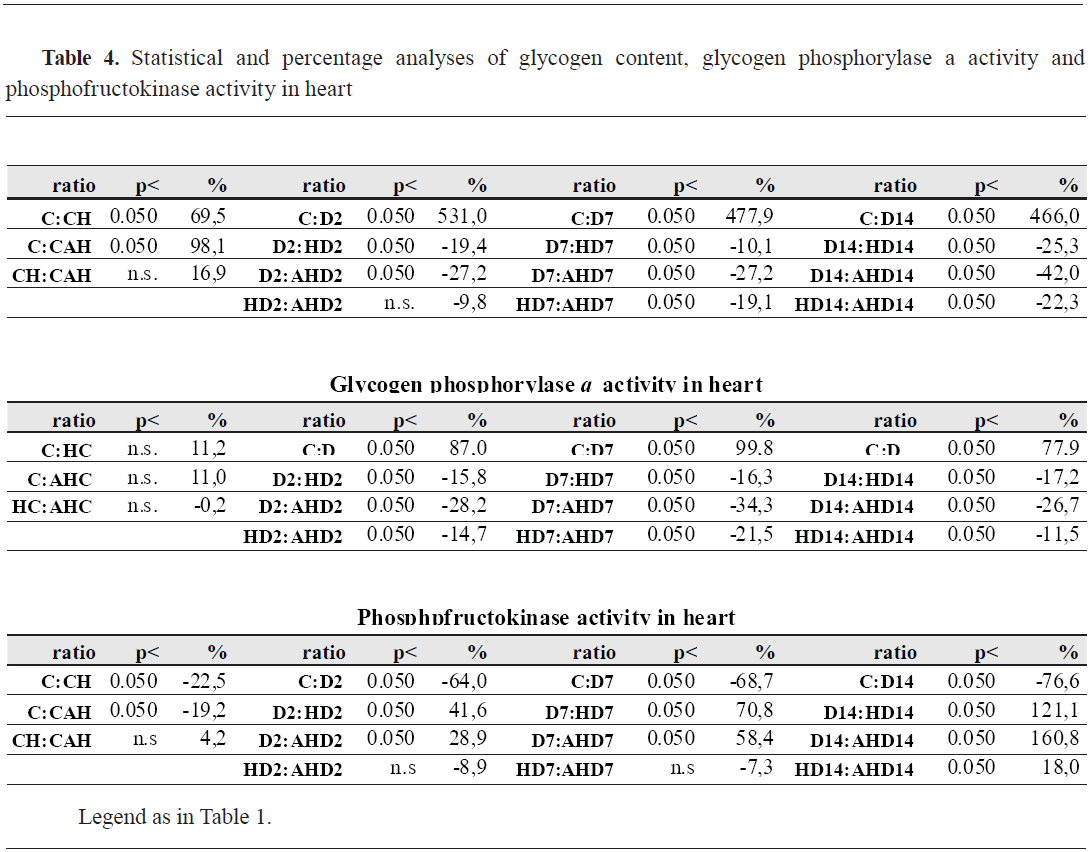
Both heat stress and ASA treatment did not caused changes in glycogen phosphorylase a activity (GPho a) in heart of control animals. Similarly to heart glycogen content, experimental diabetes (regardless of duration) caused an evident increase of GPho activity (about 80-100%), HP of diabetic animals resulted in significantly lower GPho activity (16%) and ASA treatment of HP-diabetic animals caused additional decrease of GPho activity (about 30%).
Both HS and ASA treatment caused significant decrease in phosphofructokinase activity (PFK) in heart of control animals (about 20%). Experimentaldiabetes (regardless of duration) caused an evident decrease of PFK activity (about 65-75%). There was significant and gradual increase of PFK activity in HP-diabetic animals (from 41 to 121%), and - the changes caused by ASA-pretreatment were almost the same.
DISCUSSION
As a follow up of our previous work (
24, 25), where metabolic changes in pancreas and liver were investigated, we continued our work with a heart as a tissue, and effect of heat stress with or without ASA pretreatment in the control and diabetic rats, since the heart has specific carbohydrate metabolism.
First of all, we found about 2.9-fold increase of HSP70 protein level in the heart 24 h hours after the HS and additional production of HSP70for about 6.8 fold when ASA was given as a pretreatment to acute HS. Our assumption is this is a direct consequence of the elevation of the rectal temperature for about 3.7 °C after the given heat stress (45 min, 41±0.5 °C) (
24). In addition to this, we have already observed that more intensive rise of the rectal temperature, which was about 4.5 °C higher than in control rats when ASA treatment was given before HS (
24). Namely, sublethal heat stress (HS) is known to induce synthesis of HSPs which plays an important role in the cell’s ability to survive against more intensive stressors (
2). In this sense, the production of HSP72 in the myocardium, which occurs 24 h following whole-body hyperthermia, is accompanied with protection against ischemia– reperfusion injury (
26).
Beside the physiological induction of HSP, there are search for an alternative stimulus, which will increase the production of stress proteins. In this sense, aspirin-plus-heat treatment (100 mg/kg ASA, 60 min prior to their placement to heat stress), according to Fawcett et al. (
7), resulted in 3–4-fold higher levels of HSP70 mRNA in different rat’s tissues, relative to those seen with heat treatment alone. On the other side, aspirin treatment alone (in the absence of heat) did not alter HSP70 protein expression, both in vivo (
7) and in vitro (
17).
Concerning carbohydrate metabolism related parameters, the most evident changes was glycolysis, which was less intensive in ASA+HS treatment. The changes that we observed in the heart carbohydrate metabolism are quite different from those in liver (
25) confirming the specific metabolism of heart as a tissue.
Furthermore, our results showed that STZinduced diabetes caused а decrease of HSP70 protein level in heart, same like in the liver of diabetic rats (
24). According to Hooper et al. (
3), protein glycation and oxidation, impaired stress response, protein aggregation and inflammation, free radical formation, which can be assessed in various organs and tissues with low levels of HSPs, might be a clue to the etiology of the disease itself. Our result is in accordance with Kurucz et al. (
27) indication of decreased HSP protein level in the heart, liver and muscle. Our results show that experimental diabetes was also manifested generally with stimulation of the glycogenetic processes and inhibition of glycolytic processes in the heart, since it is known that diabetic heart, the initial steps of insulin signaling and glucose transport are defective (
28).
Glycogen deposition in heart of diabetic rats exists despite the fact that glycogen synthase expression is not increased but rather suppressed due to the inability of insulin to induce its activity (
29). Also, as one of the major regulators of cardiac energy metabolism, AMP activated protein kinase which indirectly or directly regulates the activity of enzymes from carbohydrate and lipid metabolism (
30). Its activation initiates redistribution of GLUT4, and in some ways favors myocardial glucose transport without the presence of insulin (
31).
Concerning heat stress and experimental diabetes, it is important to stress that heat preconditioning (24 h hours after the HS), first of all, causes increaseof the HSP70 protein level in the heart of diabetic rats, thus reached control values. Increased HSP72 and HSP25 content in the heart of heat- stressed diabetic animals was found in the heart, kidney and liver, compared with the same tissues from heatstressed but nondiabetic animals (
32). The evidence suggests that HSP70, as the primary mediator of cardioprotection, might be a direct correlation between the amounts of HS –induces HSP70 and the degree of myocardial protection in the rabbit (
33). According to Joyeux-Faure et al. (
2) HS is able to induce a delayed myocardial protection (occurring 24-48 h after the HS) against ischaemia-reperfusion injury by induction of different chemical signals, as well as HSPs.
In this sense, we hypothesized that increased HSP70 protein level in heart enable the organism to resist the adverse effects of stress, such as diabetic disturbances in carbohydarate metabolism of heart. Namely, we found that HP-diabetic rats showed less intensive changes than diabetic rats from room temperature. Compared to diabetic controls, HP-diabetic rats have significantly lower Glk and G6P content and GPho and HK activity, as well as higher Glu content and PFK activity. It is important to stress that HP has a tendency to reverse the estimated parameters. Some similar investigations of Marber et al. (
33) showed that heat stress enhances the ability of rabbit papillary muscle to use pyruvate, but not glucose, and that induction ofthe HSP72 is responsible for the differences seen in contractility of the muscles.
Our consideration is that increased synthesis of cytoprotective HSP70 speeds up recovery from additional stress, which in our results is manifested with moderation of the diabetic disturbances in carbohydrate metabolism. Nevertheless, some opposite conclusions rise from results of Joyeux- Faure et al. (
2). Namely, in a model of insulindependent diabetic rats, they have investigated the effects of prior heat stress on the infarct size in the isolated heart and on the myocardial heat stress protein (HSP) 72 synthesis. Finally, they found that heat stress is unable to protect myocardium infarction in STZ-induced diabetic rats.
Our results showed that HS caused increased of HSP70 protein level both in control and in diabetic rats. Also, ASA pretreatment rats caused additionalelevation of HSP70 level in control rats. Our results showed that ASA-pretreatment of HP-diabetic animals also causes additional increase of HSP70 protein level. This indicates for more intensive common effects of ASA and HP over HSP70 production and leads to a consumption that ASA, in a combination to previous HS, is a potent coinductor of HSP70 both in control and in diabetic animals.
Aspirin, by acting as a co-inducer of HSP and emphasizing HSP expression (
7), can cause a reduction of the temperature threshold of the heat shock response (
34). Some investigations made with chicken myocardial cells (
11), showed that in response to heat stress, ASA stimulated nuclear translocation of HSF-1 and HSF-3 and binding to Hsp70 HSE. Still, in the absence of HS, ASA is unable to upregulate HSP70 expression. According to Wu et al. (
9), acetylsalicylic acid protected against HS damage in myocardial cells in chickens and may be associated with elevated induction of HSP27 expression. According to Zhang et al. (
35) in the aspirin-treated myocardial chicken cells there was 11.1-fold higher HSP90 level than that in non-treated cells and this elevated level remained at the early stage of heat stress. On the other side, it was just 4.1-fold higher level of HSP90 in only heatstressed cells and these returned rapidly to a low level. Based on this, again, we hypothesized that the common effect of HP and ASA treatment over elevation of HSP70 protein level can obtain more intensive protection against diabetic disturbances in carbohydrate metabolism in heart of diabetic rats, which was manifested in our results.
Still, some of the changes can be related to the earlier findings include hypoglycemic effect of ASA in diabetic rats. According to Coe et al. (
36), aspirin therapy reduces diabetic mouse nonfasting blood glucose levels, has an ability to stimulate insulin and glucagon secretion and to provide improved glucose tolerance in control animals. However, according to Martha et al. (
37) aspirin pretreatment (10 mg/kg/day for one and two months) most obviously protect the pancreas from STZ-damage and regulates glucose levels in diabetic rats. Moreover, they found increased insulin sensitivity and reduced insulin resistance, which may indicate of involvement of IGF-1 in an insulin like pathway.
CONCLUSION
Heat preconditioning (HP) is a physiological inductor of HSP70 in the heart. Acetylsalicyl acid (ASA) given prior to HS-exposure, is a potent pharmacological HSP70 co-inductor. As a results of this, both HP and ASA-pretreatment tended to reverse most of the carbohydraterelated parameters almost the control values of intact rats. The changes are more evident by ASA-pretreatment of HS-exposed rats. Finally, both HP and ASA might protect the heart tissue against higher doses of stress.
CONFLICT OF INTEREST
The authors declare that they have no potential conflict of interest with respect to the authorship and/or publication of this article.
ACKNOWLEDGMENTS
The research was performed at the Department of Experimental Physiology and Biochemistry, Institute of Biology, Faculty of Natural Sciences and Mathematics, Ss. Cyril and Methodius University in Skopje, North Macedonia.
AUTHORS’ CONTRIBUTION
MD was main investigator, involved in all phases of work and writing; SDK was involved in creating of the research protocol; ER and IC were involved in the laboratory work; SP was a supervisor of the research work; BM- was a supervisor of the whole work, involved in all phases of work and writing.