β-lactamases are a diverse class of enzymes produced by bacteria that present a major cause for resistance to β-lactams. In this study we analysed 159 fecal samples from dairy cows, for the presence of presumptive ESBL, AmpC, and carbapenemase-producing E. coli. Phylotyping was done using Clermont phylo-typing method, targeting arpA, ChuA, and YjaA genes, along with the DNA fragment TspE4.C2. Convetional PCR method was used to confirm the presence of bla genes among 39 phenotypically confirmed ESBL producing E. coli. The results showed presence of CTX-M, SHV, TEM and OXA1 bla genes in 28 (71.79%), 1 (2.56%), 29 (74.35%), 2 (5.12%) of isolates, respectively Twenty (51.28%) isolates showed presence of both blaCTX-M and TEM genes. The strain that carried the blaSHV gene was found to carry blaTEM gene as well, while one of the strains that carried blaOXA1 gene was also carrying blaCTX-M and TEM gene. The ration between isolates and phylo-groups was as follows: 9 (23.07%) strains were assigned to phyllo-group D; 14 (35.89%) to phyllo-group B; 16 (41.02%) to phyllo-group A. Out of the 39 strains where bla genes were identified, 29 (74.35%) were categorized as multi drug resistant.
In recent years, there has been an increase in reports of antimicrobial resistance (AMR) in intensive animal production. As a result, much attention is paid on the function of antimicrobial administration and how much it influences the development of resistance in microbiota originating from food producing animals. Antibiotics are usually used in cattle for medicinal, preventive, meta phylactic, or growth-promoting goals (
1). According to various reports in the developing countries, 80% of the antimicrobials used on farm animals are for growth promotion and prevention (
1). Numerous studies have revealed that this extensive use of antibiotics may have had a significant role in the selection and spread of antimicrobial-resistance among bacteria (
2), and in the existence of antimicrobial resistance (AMR) genes in the genome of the human and animal microbiome, as well as in bacteria originating from the environment (
3). Resistant pathogenic bacteria are the main cause for recognizing antimicrobial resistance (AMR) as top ten priority of the “One Health” concept, because it affects humans, animals and the environment (
4).
To monitor the AMR prevalence in healthy animals, commensal bacteria, such as
Escherichia coli (
E. coli) is widely used as indicator for monitoring AMR on farms (
5). Moreover, since manure is used to improve soil quality, animal faeces poses as a significant threat for the dissemination of resistant bacteria into the environment and potentially to humans (
6).
For clinicians and public health officials, the resistance of commensal
E. coli to β-lactam antibiotics due to extended spectrum β-lactamases (ESBLs) is a particular cause for concern (
7). ESBLs are a class of enzymes that hydrolyze oxyimino-β-lactams including extended-spectrum cephalosporins, while excluding cephamycins and carbapenems (
8). So far, a wide range of ESBL types has been described. Although
E. coli bla genes exhibit wide genetic diversity,
blaSHV,
blaTEM, and
blaCTX-M enzyme types are the most prevalent ones (
9).
In recent years, it has become important to evaluate how different phylogenetic groups of
E. coli strains differ in their antibiotic resistance. According to phylogenetic analysis,
E. coli falls into four major phylogenetic groups (A, B1, B2, and D).
E. coli strains from phylogenetic groups B2 and D are often more virulent, but less resistant to antimicorbials, whereas most commensal strains belong to groups A and B1 (
10). The latter ones are less virulent but possess higher resistance rate (
10).
The commensal
E. coli strains isolated from animal faeces may be affected by geographic region or the purpose for which animals are being kept (dairy or beef), as well as by the antimicrobial treatments that animals are receiving, if any. The improper use of antibiotics by farm owners should not be neglected as well, as a crucial link in the complete antimicrobial resistance chain. Additionally, commensal
E. coli can acquire some virulence genes from other bacteria through horizontal gene transfer, resulting in different pathovars causing clinically and epidemiologically distinctive diseases (
11). Therefore, it is important to have knowledge of the antimicrobial resistance patterns of commensal
E. coli originating from animal’s faeces and their phylogentic group belonging. For that purpose, we conducted this research, to screen the presence of
bla genes and phylogenetic group belonging of ESBL-producing commensal
E. coli strains, isolated from faecal samples originating from dairy farms in the Municipality of Debar.
MATERIAL AND METHODSSample collectionFor the purpose of this study, 159 faecal samples were taken from 34 dairy farms from the territory of Debar Municipality, during the period from June 2019 to February 2020. The farms were chosen by their location, to be able to cover a major part of the Municipality of Debar.
Isolation and identification of ESBL E. coli
All 159 faecal samples from dairy cows, were analyzed for presence of presumptive ESBL, AmpC, and carbapenemase-producing
E. coli according to the EU Reference Laboratory for Antimicrobial Resistance (EURL-AMR DTU) and the Directive
2013/652/EU (
12, 13). At the initial step, one g of faecal sample was enriched with Buffered Peptone Water (BPW) (Merck, Germany) in a ratio 1:10, and then incubated for 18 to 22 hours at 37±1 °C. Than one loopful (10 μl loop) from the enriched sample was subcultured over the surface of MacConkey agar (Oxoid, UK) supplemented with 1 mg/L cefotaxime (CTX) by streaking and incubated at 44 °C+/-0.5 °C for 18-22 hours. Presumptive ESBL/AmpC producing
E. coli lactose fermenting enterobacterales exhibited red/purple colonies on MacConkey plates. To confirm the presence of
E. coli, a colony from MacConkey+Cefotaxim plate, was cultured on TBX medium. Green colonies growth on TBX plates were considered as presumptive
E. coli.
DNA extractionGenomic bacterial DNA isolation was performed from fresh culture on a pure bacterial isolate from nonselective TSA agar by suspending a loop full of colonies in 990 μl UltraPure DNase/Rnase free distilled water (Invitrogen, USA). The bacterial suspension was than incubated 15 minutes at 100 ºC in Thermo block (MRC, Israel) without shaking. The resulting thermolysate was then centrifuged at 18,000 g/5 min. (Hettich, Germany), after which the supernatant was transferred in new tubes and kept at -20 ºC until further analyses.
β-Lactamase genes confirmationConventional PCR method was performed in order to detect
blaCTX-M,
blaTEM,
blaSHV,
blaOXA1, and
blaOXA2 genes in isolates with an ESBL phenotype (
14). PCR, assay was performed using primers displayed in
Table 1. PCR protocol (Qiagen, Netherlands) consisted of 30 cycles (30 s of denaturation at 94 ℃, 30 s of annealing at temperatures as shown in
Table 1 and 60 s of extension at 72 ℃) after one step of 5 min at 94 ℃ (
14). PCR products were analyzed in 2% agarose gel (Merck, Germany) containing 25 μg of ethidium bromide (Merck, Germany) in tris-EDTA buffer, and the gel was photographed under ultraviolet illuminator using gel documentation system ChemiDoc MP Imaging System (Bio-Rad, USA) stain-free technology imaging, analyzing and documentation of gels.
The Clermont Escherichia coli phylo-typing methodologyIsolates that exhibited standard
Escherichia coli phenotype (such as lactose +, green colonies on TBX plate) were tested using the quadruplex approach following the protocol as described in (
15). First, the isolates were subjected to quadruplex PCR. Each strain is assigned a quadruplex genotype based on the presence or absence of the four genes. The isolate was then allocated in a phylo-group based on the quadruplex genotype obtained by scoring the presence/absence of the genes in the order arpA/chuA/yjaA/TspE4.C2, as given in
Table 2.
Primer sequences and PCR conditions for the new phylo-group assignment method are presented in
Table 3.
All PCR reactions were carried out in a 20 μl volume containing 2 μl of 10X buffer (provided with Taq polymerase), 2 mM each dNTP, 2 U of Taq polymerase, 3 μl of bacterial lysate or 2 μl of DNA (at about 100 ng ml
-1) (Applied Biosystems, USA) and the relevant primers (Invitrogen, USA). The primers’ concentration in the PCR reaction was 20 pmol for all primers, except for AceK.f (40 pmol), ArpA1.r (40 pmol), trpBA.f (12 pmol), and trpBA.r (12 pmol). Denaturation was performed 4 minutes at 94 °C, followed by 30 cycles of 5 seconds at 94 °C and 20 seconds at 57 °C (group E) or 59 °C (quadruplex and group C), and a final extension step of 5 minutes at 72 °C. ArpAgpE.f and ArpAgpE.r and trpAgpC.f and trpAgpC.r were the primers used for the allele-specific phylo-groups E and C PCRs, respectively (
17). As an internal control in E- and C-specific PCR reactions, the primers trpBA.f and trpBA.r were used (
15).
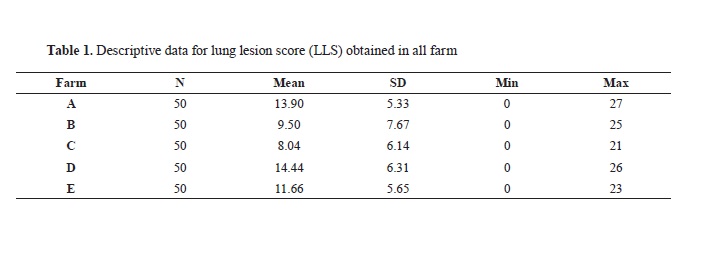
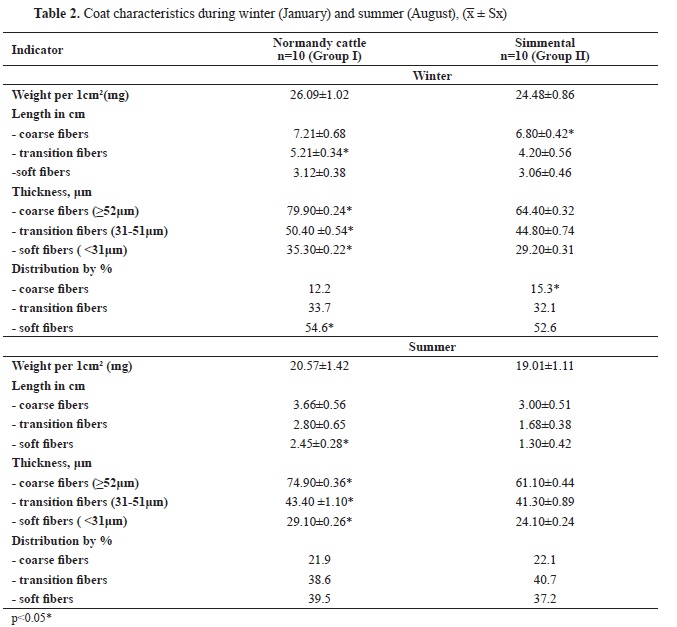
RESULTSOut of the 159 faecal samples from dairy cows, 39 (24.52%) were phenotypically confirmed as presumptive ESBL-producing
E. coli. The presence of
bla genes in isolates with the ESBL phenotype was confirmed by molecular analyses in 38 out of 39 (97.43%). The results showed presence of 28 (71.79%), 1 (2.56%), 29 (74.35%), 2 (5.12%) for CTX-M, SHV, TEM and OXA1
bla genes, respectively. No OXA2
bla gen was detected. Twenty strains (51.2%) showed presence of both
blaCTX-M and TEM genes, while one of the strains (2.56%) carrying
blaSHV gene was found to have
blaTEM gene as well. One of the two strains positive to
blaOXA1 gene was found to be carrying
blaCTX-M and
blaTEM gene, while the other strain was carring
blaOXA1 and
blaTEM.
Regarding the results from phylo-typing, all strains were assigned to three phylo-groups out of seven. The data indicated that most prevalent phyllo-group is A (41.02%), followed by B1 (35.89%) and D (23.07%).
The distribution of
bla genes in relation to the phylogenetic group is given in
Table 4. From the isolates carrying
blaCTX-M gene 12 (42.85%) belonged to phylo-group B1, followed with 11 (39.28%) isolates in phylo-group A and 5 (17.85%) in phylo-group D.
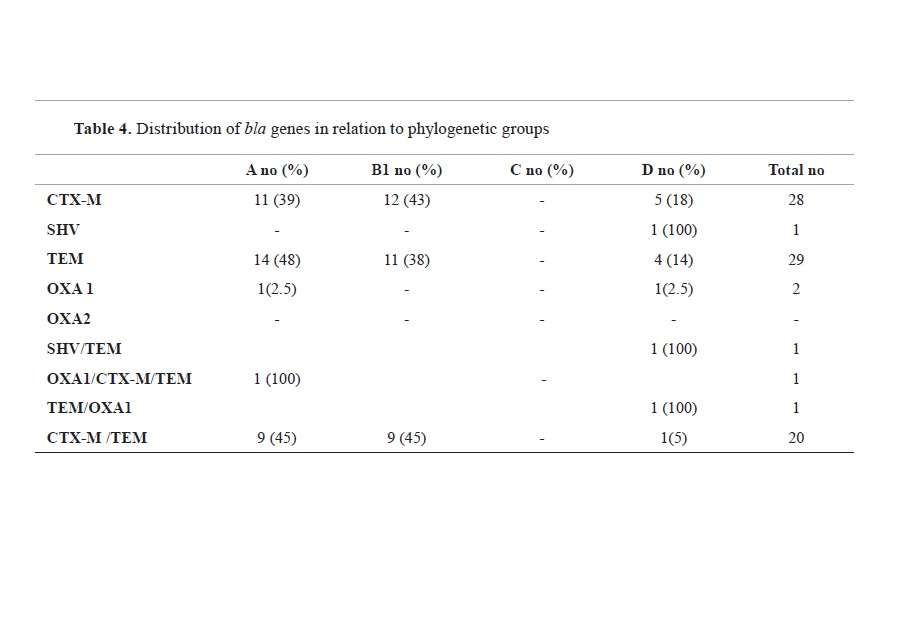
Almost half of the isolates (48.27%) carrying
blaTEM gene, were assigned to phylo-group A, while 37.93% belonged to phylogroup B1 and 14% were assigned to phylo-group D. The one isolate in which
blaSHV gene was detected, belonged to the phylo-group D, while the two isolates carrying OXA1 gene were assigned to phylo-group A and D, each.
According to the antimicrobial susceptibility testing of all isolates with the use of micorbroth dilution method (data not shown), 29 (74.35%) strains were categorized as multidrug resistant (MDR). Out of the 29 MDR isolates, 48.27% belonged to phyllo-group A, followed by groups B1 and D with 27.58% and 24.13%, respectively.
DISCUSSION
ESBLs are plasmid-encoded, meaning that these resistance determinants are prevalent in our environment and may be easily transmitted from one organism to another. The significant prevalence of these ESBL producers in animal fecal samples supports the hypothesis that animals might become infection sources or even reservoirs (natural persistent sources of infection), aiding in the spread of these bacteria. The findings and supporting data given here imply that dairy cattle farms are a substantial source of ESBLs producing
E. coli that play an important role in resistance dissemination across the ‘One Health’ concept. In our survey, the ESBL phenotype was found in 24.52% of all commensal
E. coli isolates. Similar results 24.6%, were obtained in faecal samples from intensive swine farms in Portugal (
17) and in wild ducks’ faeces from Bangladesh (
18). However, these results are lower than the ones reported for ESBL phenotype in dairy cattle from China (45.9%), Egypt (46.6%) and Nigeria (63.2%) (
19, 20, 21). Similar results as in this survey were obtained in a study that involved an outpatient setting in Nepal, where ESBL confirmed phenotype was 25.8% (
22), in uropathogenic
E. coli isolated from outpatients in Iran (
23) and in rectal samples from healthy infants, where ESBL
E. coli was confirmed in 33.3% (
24). Numerous studies have reported a high prevalence of ESBL producing
E. coli in environmental samples, especially from farm environments. In a study on the diversity of ESBL producing
E. coli in poultry farm environment, ESBL phenotype was confirmed in 100% of soil samples, in rinse water and run off water (81% and 79%, respectively) and 60% in dust (
25). These studies illustrate identifiable epidemiological cycles between particular reservoirs, such as cattle (and meat), people in general and clinical populations.
The first reports of
blaCTX-M-carrying bovine faecal
E. coli isolates in American livestock came in 2010 (
26). The TEM and SHV genes predominated in the earliest ESBL cases, but more and more CTX-M cases are now being reported from a number of countries, including Africa, India, Iran, and industrialized nations like France, Canada, and the UK (
27). The
blaCTX-M-1 gene was found to be the most prevalent in two separate independent studies that used faecal samples from various barns to search for the most common
bla genes in
E. coli. The first study involved Thoroughbred race horses in Ontario, Canada (
28), and the second involved race horses in Korea (
29). The poultry industry revealed similar results for other Enterobacteriaceae family members, specifically S
almonella enterica and
Escherichia coli, respectively. Both strains had high levels of
blaCTX-M-1, which originated from the same source: the gastrointestinal tract of Dutch broilers (
30).
In terms of the prevalence of
bla genes, we found that 97% of the strains carry at least one of the targeted bla genes; however, thorough genotype analyses revealed that
E. coli isolates carried a variety of ESBL
bla genes, with
blaTEM and
blaCTX-M being the two most common. The reported findings are consistent with a crosssectional study on outpatient departments (OPD) and intensive care units (ICU), which found that 25.8% of strains were phenotypically confirmed to be ESBL
E. coli, with the most prevalent type being
blaCTX-M, which was followed by
blaTEM (29.2%) and
blaSHV (12.5%) (
23).
Despite the fact that isolates displayed phenotypic traits toward a wide range of cephalosporins, which can be explained by the potential presence of other ESBL genes, in our results only one isolate did not show presence of any of the targeted
bla genes. Similar data were obtained in a study that investigates ESBL phenotypes and genotypes among ESBL-positive isolates from outpatients (
23). Increased distribution of the ESBL genotype
blaCTX-M in humans, animals, and environmental sources reveals a crucial concern in the management of infectious diseases (
31).
The data obtained in this study have been analyzed regarding the coexistence of various β-lactamase genes within the same strain and their interactions. The results showed that 2.6% of the strains that carried the
blaSHV gene, were found to be positive for the
blaTEM gene, and 69% of strains demonstrated presence of both the
blaCTX-M and
blaTEM genes. It was discovered that one of the two strains (5.4%) that were positive for the
blaOXA1 gene also carried the
blaCTX-M gene. These findings are in correlation with the data from numerous similar studies in both human and veterinary medicine (
17, 22, 23, 32).
According to our findings, 23% of the isolates were in group D, 36% were in group B1, and 41% belonged to phylo-group A. The classification of commensal
E. coli into the A and B1 phylogroups was as expected from the beginning of this study and supported by findings from other studies. For example, a study that evaluates the genotyping diversity of
E. coli from untreated, healthy dairy cows confirmed that the isolates belonged to phylogenetic groups A, B1, B2, and D, with the majority of isolates belonging to B1 and then A (
33). Additionally, the phylogenetic analysis of
E. coli from geographically distinct human populations revelaed that strains from phylogenetic groups A (40%) and B1 (34%) were most prevalent, followed by strains from phylogenetic group D (15%) (
34).
The most common commensal
E. coli strains are found in group A, while the most virulent extraintestinal strains are primarily found in group B2 and to a lesser extent, group D (
35). Many scientists have looked at the distribution of the main phylogenetic groups among the
E. coli strains found in humans and animals. While human groups A and B1 are commensal strains, groups B2 and D are frequently designated for extraintestinal pathogenic
E. coli (
31). Moreover, our findings are consistent with those of a study on domestic animals, specifically dogs and their owners, in which two groups of isolates were assigned to phyllo-groups to evaluate their phylogenetic relationships. Findings show that the isolates had similar genotypes, with phylo-group A accounting for the majority of cases (55% of dogs and owners) and being the most prevalent (
36). The A and B1 groups are more frequently associated with commensal strains on one of the phylo-groups. In other animals and various studies, the assignment of
E. coli ESBL strains was made, for instance, it was discovered that group B1 was more prevalent in isolates of
E. coli in healthy food-producing animals. While group A was discovered to be more common in pigs and chickens (
17, 37), group B was found to be more common in cattle (
36).
CONCLUSIONSIn conclusion, our survey demonstrated the overall situation of antibiotic resistance in faecal
E. coli isolates from the dairy farms in the Municipality of Debar. The findings suggest that dairy cows might be a significant reservoir of resistant
E. coli, thus posing a substantial risk to human health. It is due to the fact that dairy cattle have a direct connection to environmental variables, particularly water, and that contaminated water significantly contributes to the spread of β-lactamase producing
E. coli in humans. The high occurrence of MDR ESBL-producing
E. coli in this survey is a cause for concern, emphasizing the importance of following rules concerning the use of antibiotics and growth promoters in food-producing animals.
CONFLICT OF INTERESTThe authors declare that they have no potential conflict of interest with respect to the authorship and/or publication of this article.
ACKNOWLEDGMENTSThis research was part of the project financed by Faculty of Veterinary Medicine-Skopje, Code FVM-IPR-01 (Decision no. 0202/2090/3, from 3.12.2019) with the title “Antimicrobial resistance of commensal
Escherichia coli in Republic of Macedonia”. The authors would like to thank colleagues from the Laboratory of food and feed microbiology and Laboratory for molecular food analyses and GMO at the Food Institute at the Faculty of veterinary medicine in Skopje, for their cooperation and contribution to this study.
AUTHORS’ CONTRIBUTIONSMK conceived the study, did the experimental examinations and wrote the manuscript. MK and MP contributed with the collecting of the raw milk samples. BSD, MRM and MP contributed to the microbiological and molecular analyses. MRM, DJ, BSD, AD and KB contributed to the study design. MP and AD contributed to data analyzing. KB, MRM and DJ supervised the study, gave critical revision and contributed to the final version of the manuscript.