The main part of serotonin in the body is synthesized and released by a certain type of enteroendocrine cells in the intestinal mucosa called enterochromaffin cells. The scarce qualitative and quantitative data on enterochromaffin and serotonin-positive mast cells in porcine extrahepatic bile ducts and gallbladder, motivated us to undertake the present study. The aim of this study was to determine the localization and density of serotonin-positive cells in the wall of the extrahepatic bile ducts and gallbladder in pigs. An immunohistochemical method was used to identify enterochromaffin cells and determine their percentage relative to the total number of endocrine cells labeled with chromogranin A. Serotonin-positive mast cells were identified after tryptase staining of serial sections. The endocrine function of mast cells was demonstrated by chromogranin A immunolabeling. The highest number of enterochromaffin cells were found in the intramural part of the ductus choledochus, followed by the papilla duodeni major, extramural part of the ductus choledochus, ductus hepaticus comunis, ductus cysticus, and gallbladder. In all parts of the extrahepatic bile ducts, the highest number of mast cells was found in the muscle layer, followed by the serosal layer and the propria. The expression of serotonin in the enterochromaffin cells of the biliary glands and in the mast cells of the analyzed organs suggests a possible synthesis of serotonin, which probably regulates physiological and pathological processes.
Serotonin (5-hydroxytryptamine, 5-HT) is mainly produced by enterochromaffin cells (ECs) (
1, 2, 3) with tryptophan hydroxylase 1 (
4, 5, 6). The intrinsic and extrinsic afferent nerves do not reach the gut lumen and are therefore not directly elicited by the lumen contents. Hence, EC cells react to chemical and mechanical stimuli (
7), activating intrinsic and extrinsic afferent nerves (
8).
Data on the presence of single serotonin-positive cells in the mucous layer of the gallbladder (GB) in goats date from 1978 (
9). Sand et al. (
10) observed serotonin expression only in some epithelial cells on the surface epithelium of the gallbladder and the major duodenal papilla (PDM) in pigs. They have not detected serotonin expression in the secretory cells of these tissues nor in extrahepatic bile ducts (EHBD). These findings have prompted the question if it is possible for serotonin immunopositive cells to exist in the intramural biliary glands of the porcine gall bladder,
ductus cysticus (DC),
ductus hepaticus communis (DHC),
ductus choledochus (DCH), and
papilla duodeni major. In our previous study (
11), chromogranin A and serotonin-positive cells were qualitatively detected in porcine gallbladder glands and their draining ducts. No other serotoninpositive structures were identified.
Chromogranin A is the specific immunohistochemical marker for neuroendocrine cells in normal and pathological conditions, having a crucial role in the synthesis of other biologically active peptides, hormones and neuropeptides (
12).
The main sources of 5-HT in the body are the mucosal ECs and enteric neurons of the gastrointestinal tract (
13, 14, 15). 5-HT released from the myenteric plexus neurons can contribute to both fast and slow excitatory postsynaptic potentials (
16) by 5-HT3 receptor inhibition (
17, 18). In the gastrointestinal tract, serotonin regulates peristalsis, secretion, vasodilation, and sensation of pain or nausea via variety of 5-HT receptors: 5-HTI (5-HT1A, 5-HT1B, 5-HTID, 5-HTIE, and 5-HT1F), 5-HT2 (5-HT2A, 5-HT2B, and 5-HT2C), 5-HT3, 5-HT4, 5-HT5 (5-HT5A, 5-HT5B), 5-HT6 and 5-HT7. Some of them such as 5-HTR1, 5-HTR2, 5-HTR3, 5-HTR4, and 5-HTR7 (
19, 20, 21).
Several studies (
22, 23, 24) reported the action of serotonin and the 5-HT2 antagonist ketanserin on the human hepatic vessels. For example, it was demonstrated that it can stimulate hepatic artery contraction in patients with liver cirrhosis (
24). Serotonin has been shown to regulate biliary tree growth via 5-HT1A and 5-HT1B receptors in a bile duct-ligated rat model for cholestasis (
25). Proliferation of the bile duct epithelial cells (BDEC) was significantly inhibited by agonist activation of 5-HT1A (8-OHDPAT) and 5-HT1B (anpirtoline). Marzioni et al. (
25) also claimed that biliary epithelial cells can secrete serotonin. The authors hypothesized the existence of a self-directed BDEC antiproliferative circuit regulated by serotonin. Selective serotonin reuptake inhibitors (SSRIs) such as citalopram are associated with cholestasis in humans (
22, 26). The mechanisms by which SSRIs contribute to cholestasis are not elucidated yet. Based on the fact that BDECs express 5-HT1A and 5-HT1B receptors, serotonin may regulate bile flow. Some authors established the role of serotonin in promoting liver fibrosis by activating myofibroblasts (
27, 28).
Omenetti et al. (
29) have demonstrated that bile duct cholangiocytes play a crucial role in hepatic homeostasis and immunity and that they can express tryptophan hydroxylase 2 (TPH2) and synthesize 5-HT that can suppress cholangiocyte proliferation through a negative feedback mechanism. However, in response to biliary injury, 5-HT stimulates myofibroblasts in producing TGFβ1 which activates cholangiocyte proliferation.
Mast cells, apart from ECs, are considered another source of serotonin in the gut and have been studied mainly in laboratory animals and humans (
30, 31). We were unable to find data on the presence and distribution of serotonin-positive mast cells in the EHBD, major duodenal papilla, and gallbladder of the pig.
Data on the ability of human mast cells to synthesize serotonin are controversial. Buhner and Schemann (
31) claimed that human mast cells in the mucosal layer of the intestine cannot synthesize 5-HT, contradicting other reports (
30, 32, 33). The possible role of mast cell serotonin can be explained in different ways. It can influence the function of various immune cells. For example, mast cell 5-HT is a chemoattractant for eosinophils (
34, 35). Dendritic cells and monocytes can be activated by serotonin via the 5-HT3, 5-HT4, and 5-HT7 receptors to secrete interleukins (
36, 37, 38) and to promote inflammation (
39, 40).
The foundations of the current study were: the important role of serotonin synthesized by intestinal ECs and mast cells established mainly in laboratory animals and man; the scarce information on the distribution of ECs and serotonin-producing mast cells in the wall of the extrahepatic bile ducts (EHBD), major duodenal papilla (PDM), and gallbladder (GB) in the domestic pig as preferred experimental species for various morphological, physiological and biochemical studies (
41, 42); and the bile ducts related surgeries (
43).
The aim of this study was to determine the localization and quantitatively characterize serotonin immunopositive cells in the
ductus hepaticus comunis,
ductus cysticus, extramural and intramural part of the
ductus choledochus,
papilla duodeni major, and the gallbladder in the pig.
MATERIAL AND METHODSAnimalsSix male clinically healthy intact pigs (Bulgarian White × Landrace cross cross) fed the same diet at the age of 6 months (92–100 kg) were used. All animals were given time to acclimate to the new environment before slaughtering. They were supplied under the Scientific Project No. 13/2017, Faculty of Medicine, Trakia University, Bulgaria. All procedures were in accordance with Ordinance 20 from 01.11.2012 on the minimum requirements for the protection and humane treatment of experimental animals and the requirements for facilities, breeding, and/or supply (Directive 2010/63/EU of the European Union Parliament and the Council - September 22, 2010, for the protection of animals used for scientific purposes). The study was approved by the Ethics Committee of the Bulgarian Food Safety Agency with license № 174.
Tissue samples were taken from the following anatomical structures: the common hepatic duct (near the junction with the
ductus cysticus), the middle segment of
ductus cysticus, the middle segment of
ductus choledochus, intramural part of
ductus choledochus (DCHI), and the neck of the gallbladder (
collum vesicae biliaris). They were collected in the slaughterhouse immediately after slaughtering and were fixed in 10% aqueous formalin.
Histological methodsFormalin-fixed tissue samples from each animal were embedded in paraffin and cut into tissue sections of 5 μm thickness. They were mounted on glass slides, deparaffinized, and rehydrated. Some samples were first stained with hematoxylin and eosin to exclude pathological changes. Then, serial sections were processed immunohistochemically to determine chromogranin A, tryptase, and serotonin reactivity.
Immunohistochemical assessment of chromogranin A, tryptase, and serotonin immunoreactivitySerial tissue sections were washed in 0.1 M PBS and placed in 1.2% hydrogen peroxide and methanol for 30 min. Antigen retrieval was performed in buffer (pH 9.0) for 20 min. Between these steps, sections were washed with EnVisionFlex Wash Buffer, then incubated for 12 hours at 4 °C with 3 antibodies: monoclonal mouse anti-human serotonin (clone 5HTH209), DAKO, chromogranin A rabbit antibody (PA 0430) DAKO, and monoclonal mouse antihuman mast cell tryptase (MBS9510697). The sections were incubated with an EnVision detection system (DAKO) for 24 h at 4 °C. The immune reaction was displayed with diaminobenzidine. Serial sections were sequentially stained with chromogranin A, tryptase, and serotonin.
Negative control was created by the replacement of primary antibody with PBS.
Statistical analysisEndocrine cell density was determined by counting the number of serotonin and chromogranin A positive secretory cells per microscopic field X100 as well as per gland cross-section. The number of tryptase, chromogranin A, and serotonin-positive mast cells was counted on a field of view X200 using a system of a light microscope (LEICA DM1000), digital camera (LEICA DFC 290), and quantitative analysis software (LAS V4.10.0 2016). Morphometric data were statistically processed by GraphPad Prism 6 for Windows (GraphPad Software, Inc., USA), one-way ANOVA and Tukey-Kramer post-hoc test. P-values less than 0.05 were considered statistically significant. Data are presented as mean ± SD (standard deviation).
RESULTSSerotonin immunoexpression in glandular cells (Enterrochromaffin cells) of EHBD, PDM, and GBThe performed immunohistochemical reaction with an anti-serotonin antibody allowed the determining the density of serotonin-positive endocrine cells (Ser+C) in EHBD, PDM, and GB. The highest number of Ser+C was found in DCHI, PDM, the extramural part of DCH, DHC, and DC, whereas the lowest number was found in GB (
Table 1) (
Fig. 1,
2,
3). The total number of endocrine cells was determined after immunohistochemical labeling with chromogranin A (
Fig. 1,
2,
3). In EHBD, the density of Ser+C was lower than ChrA+C.
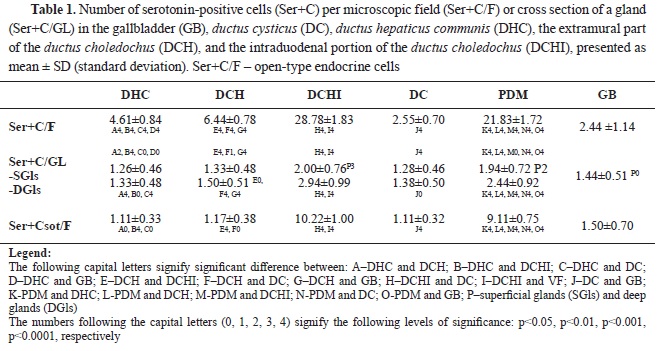
Of all EHBD segments, only DCHI and PDM had a higher density of Ser+C in the deep glandular alveoli than the superficial (p˂0.001 and p˂0.01, respectively) (
Table 1).
The percentage of Ser+C in relation to the number of ChrA+C per field of view was different for each segment of EHBD: the highest percentage was in GB–100%, followed by DHC–0.92%, the extramural part of DCH–86%, DC–83%, PDM–49%, and DCHI–48%.
The highest Ser+C of open-type (Ser+Csot) was observed in DCHI, followed by PDM, GB, and the extramural part of DCH. The lowest Ser+Csot was observed in DHC and DC.
The percentage of Ser+Cot relative to the total number of Ser+C was as follows: DHC–24%, extramural part of DCH–18%, DCHI–36%, DC–44%, PDM–42%, and GB–61%.
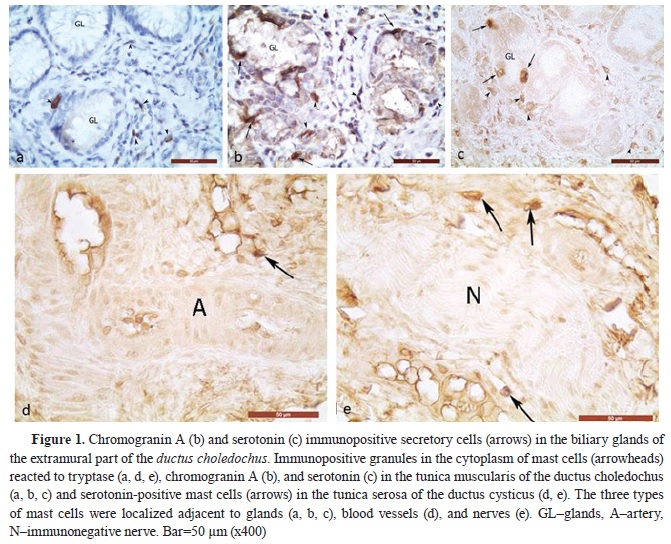
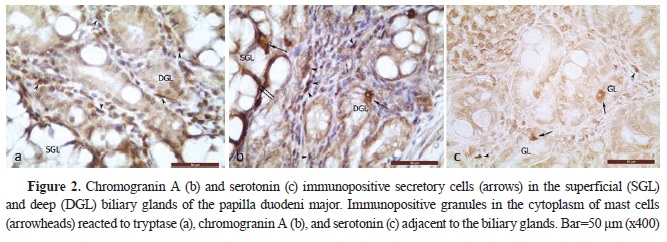
Serotonin immunopositive mast cells in EHBD, PDM, and GB
To establish the presence of serotonin-positive mast cells (Ser+MCs), an immunohistochemical visualization of tryptase-positive mast cells was performed on serial sections from the wall of the GB, DC, DHC, extra- and intramural part of
ductus choledochus, as well as from the wall of the PDM. The immunoexpression of tryptase, as the main marker for mast cells, and of chromogranin A – the marker for endocrine cells, showed conclusively that the serotonin-positive cells in the propria, musclular, and serosal layers of the examined organs are mast cells (
Fig. 1,
2,
3).
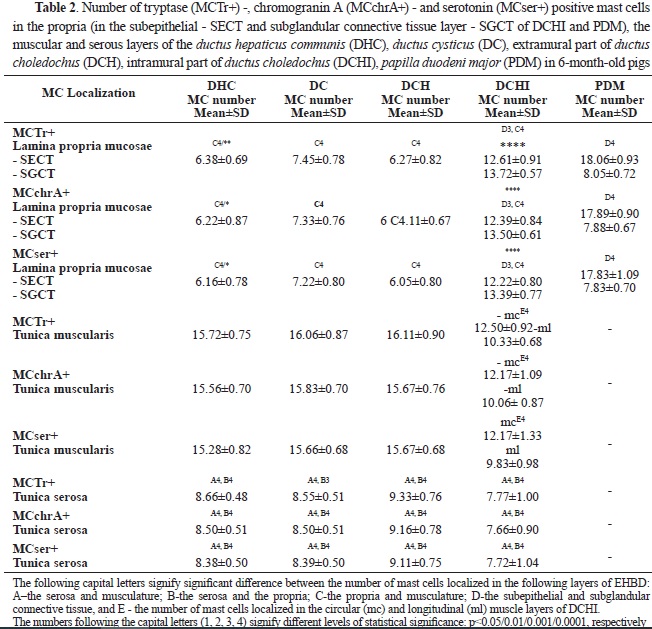
All three types of mast cells: MCstr+, MCchrA+, and Ser+MCs showed similar localization, dimensions, and immunoreactivity of their granules, being present in all layers of the examined organs.
Statistical analysis of the data found an equal amount (P>0.05) of Ser+MCs, MCTr+, and MCchrA+ in each of the layers of the EHBD and PDM (
Table 2). In all parts of the EHBD, the highest number of mast cells was found in the muscle layer, followed by the serosal layer, and the lowest number was in the propria. In PDM, a greater number of mast cells were observed in the subepithelial layer of the propria compared to the subglandular layer, while the opposite relationship was found in the intramural part of the
ductus choledochus. Mast cell number in propria was highest in PDM followed by the intramural part of
ductus choledochus and was significantly lower in other segments of the extrahepatic bile ducts. However, mast cell density in muscular and serosal layers was lowest in the intramural segment of the extrahepatic bile duct. In the circular muscle layer, mast cells predominated compared to the longitudinal muscle layer.
Ser+MCs with moderate to strong immunoreactivity were localized adjacent to capillaries, arterioles, and venules in the propria and basement membrane of the biliary epithelium of the mucosa of GB, EHBD, and PDM (
Figs. 1,
2,
3).
In the muscle layer, they were located near blood vessels, superficial and deep glands, and in the connective tissue between bundles of smooth muscle cells, with some mast cells found in close proximity to smooth muscle cells.
In the outer connective tissue layer (
tunica adventitia or
tunica serosa) of organs, Ser+MCs were observed most frequently in the adventitia of muscular-type arteries and adjacent to nerves (
Fig. 1 d, e).
Mast cells with the largest sizes were located in the subepithelial layer of the intramural segment of
ductus choledochus and PDM, followed by the muscle layer of the EHBD, and the serosal layer. In the propria of DHC, DCH, and DC, mast cells were of the smallest size (
Table 3).
In GB, most of the mast cells were found in the propria, followed by those in the muscular layer, whereas the lowest number was observed in the
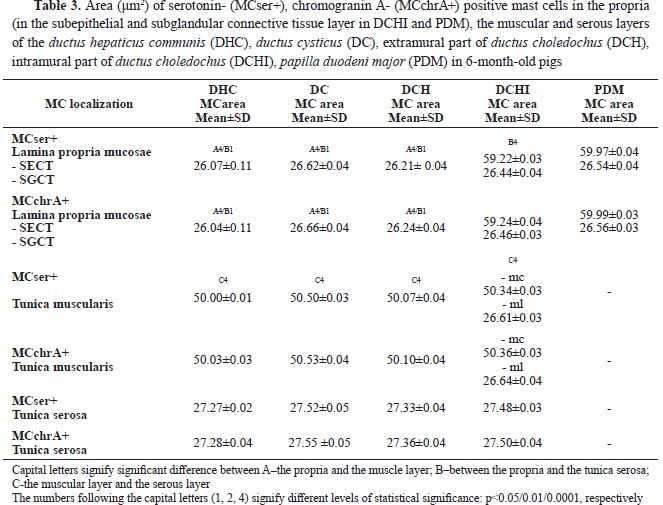
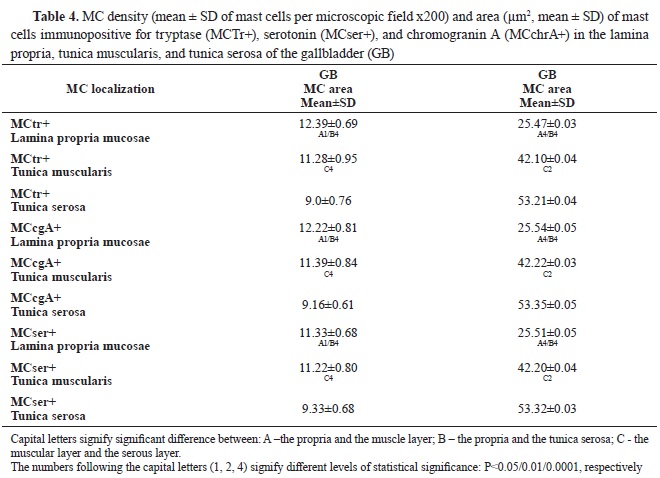
serosal layer (
Table 4). Compared with the EHBD, the number of mast cells in the propria of the GB was significantly higher, while their number in the muscular layer was lower. In the serosa of EHBD and GB, the number of mast cells showed close values.
In the gallbladder, MCTr+, MCser+, and MCchrA+ with the smallest area were observed in the propria. Their area was larger in the muscle layer, and largest – in the subserosa (p˂0.001) (
Table 4).
Compared to the EHBD, the area of mast cells in the serous layer of the GB was significantly larger. The area in the propria and muscle layer was similar to EHBD.
DISCUSSIONThe present study provided original data on the density of serotonin-expressing cells in porcine DC, DHC, DCH, PDM, and GB. Serotonin sources were immunohistochemically localized in the glandular epithelial cells (EC) and mast cells of the organ’s walls. The localization of well-defined serotonin immunoreactivity in some of the secretory cells of the biliary glands showed that EC was present in all organs that were the subject of this study. This was confirmed by immunopositivity of the specific marker for neuroendocrine structureschromogranin A, found in the secretory cells. The presence of this glycoprotein established by our study, proved that glandular epithelial cells and mast cells in porcine EHBD, PDM, and GB can synthesize hormones and neurotransmitters (
14). The current study complemented the data from the studies of Sand et al. (
10) who observed serotonin expression only in single epithelial cells in the surface epithelium of the gallbladder and major duodenal papilla in pigs, whereas no expression was observed in the secretory cells of the glands. The current study has also complemented our previous report on the qualitative presence of serotoninpositive secretory cells in the gallbladder`s glands and its excretory duct. The presence and density of enterochromaffin cells in the glands of the porcine DHC, DCH, and PDM were observed for the first time.
These findings are adding to the scientific reports on the presence of serotonin-expressing cells in the gallbladder and bile ducts in humans and pigs. Endocrine cells similar to ECs in the intestinal mucosa, were described in the human gallbladder by Gilloteaux et al. (
44). In our previous study (
11), the localization of chromogranin A and serotonin-positive cells in the iglands of the gallbladder and its excretory duct, was established without quantitative analysis. However, there is a lack of data on the localization of ECs in the glands of the extra- and intramural part of the DCH and PDM in these species, including the pig.
ECs are a type of intestinal endocrine cells (EECs) found in the small and large intestine segments. (
45, 46). The serotonin-positive cell localization in the porcine EHBD and GB in the current study is similar to other reports analyzing intestines where the cells were either from an open or closed type (
47, 48). Gustafsson et al., 2006 established that ECs with their basal extensions contact with neurons (
20). Therefore, it is indicative that ECs are also localized in the glands of healthy porcine EHBD, PDM, and GB without detection of serotonin-positive neurons. Moreover, we calculated the density of serotoninexpressing cells in the different layers of the GB and EHBD, including the large duodenal papilla, and chromogranin A positive cells percentage relative to the total number of endocrine biliary cells.
The role of serotonin in the glands of porcine EHBD and GB can be explained by the morphofunctional studies on serotonin in the gastrointestinal tract in various animals and humans. Taking into account the regulatory role of serotonin on the peristalsis, secretion, vasodilation, and sensation of pain or nausea by activating 5-HT receptors on the intrinsic and extrinsic afferent nerves in lamina propria (
18), we hypothesized that serotonin-producing cells have a similar role in the pig’s EHBD, PDM, and GB.
Several authors defined variety of 5-HT receptors and their subtypes: 5-HTI (5-HT1A, 5-HT1B, 5-HTID, 5-HTIE, and 5-HT1F), 5-HT2 (5-HT2A, 5-HT2B, and 5-HT2C), 5-HT3, 5-HT4, 5-HT5 (5-HT5A, 5-HT5B), 5-HT6, and 5-HT7. Some of them (5-HTR1, 5-HTR2, 5-HTR3, 5-HTR4, and 5-HTR7) are expressed in the gastrointestinal tract within the smooth muscle, intestinal neurons, enterocytes, and immune cells (
19, 20, 22). According to Cooke (
49), the secretion of the intestinal epithelium can be initiated by intrinsic intestinal reflexes, represented by primary sensory and secretomotor neurons with perikaryons located in the submucosal ganglia. Afferent neurons are stimulated by signals from outgrowths in the lamina propria, which activate secretomotor neurons to release acetylcholine and vasoactive intestinal peptides involved in the stimulation of epithelial cell secretion. These findings in the intestine can be used to explain the similar role of serotonin established in the ECs of the porcine EHBD and GB. Neurogenic secretory responses are mediated mainly by 5-HT3, 5-HT4, and 5-HT1P receptors (
49, 50, 51, 52). Serotonin released from EC cells can also the stimulate secretion of adjacent enterocytes in a paracrine manner via 5-HT2 receptors (
50). Some authors have found that mechanical stimulation of the mucosal surface leads to the release of 5-HT. Other authors established the bactericidal effect of serotonin (
53, 54).
The possibility of serotonin synthesis by ECs in the porcine EHBD and GB allowed us to hypothesize that a metabolic pathway for melatonin synthesis probably exists, similar to ECs in the gastrointestinal tract (
55, 56). Therefore, EHBD and GB can be considered as another source of melatonin in the body. In this relation, future studies are needed to determine whether the enzymes (acetylserotonin O-methyltransferase and arylalkylamine-N-acetyltransferase) of ECs of the porcine EHBD and GB, similar to those from the stomach and intestines, are responsible for melatonin synthesis.
The established serotonin immunoreactivity in the current study showed that the pig’s EHBD and GB lack serotonin-positive nerves, which confirms the results of Sand et al. (
10). They have concluded that serotonin role in the pig is not neurotransmission, but has paracrine regulation (
10). They observed serotonin expression only in single epithelial cells of the lining epithelium of the gallbladder and large duodenal papilla in pigs, whereas no expression was detected in the rest of the EHBD. However, we have provided detailed data on the localization of serotonin expression not only in some endocrine cells in the biliary glands but also in mast cells of porcine EHBD and GB, supported by detailed quantitative analysis.
It is known that sympathetic nerves regulate vascular tone. In the intestine, they regulate local vasodilation. Motor neurons responsible for vasodilation are found in the submucosal ganglia. There are two types of reflex circuits: local circuits consisting of afferent and motor neurons in the submucosal plexus, and a circuit represented by submucosal afferent neurons contacting the myenteric plexus and submucosal vasodilatory motor neurons (
57, 58, 59).
Vagus afferent fibers in the gastrointestinal tract lead to different responses by 5-HT stimulation. Vagus effects are mediated via 5-HT3 receptors of vagal afferent nerve endings in the intestinal mucosa (
60, 61, 62). Furthermore, infusion of hyperosmotic solutions or carbohydrate breakdown products into the duodenal lumen induces serotonin release from EC cells, which via 5-HT3 receptors, stimulates vagal afferent neurons (
63).
The main sources of 5-HT in the body are the mucosa and enteric serotonergic neurons (
13, 14, 15). Serotonergic neurons in the wall of GB and EHBD were not observed in this study.
Serotonin has been shown to regulate biliary tree growth by 5-HT1A and 5-HT1B receptors in a bile-duct-ligated rat model of cholestasis (
30). Marzioni et al. (
25) found that biliary epithelial cells can secrete serotonin, hypothesizing the existence of a self-directed bile duct epithelial cells antiproliferative circuit regulated by this hormone.
Some authors established another role of serotonin in stimulating liver fibrosis by activating myofibroblasts (
27, 28). Omenetti et al. (
29) have reported the action of 5-HT in the hepatic bile ducts, stating that bile duct cholangiocytes play a crucial role in hepatic homeostasis and immunity by expressing TPH2 and by producing 5-HT which suppresses cholangiocyte proliferation through a negative feedback. However, in biliary injury, 5-HT stimulates myofibroblasts in the production of TGFβ1 which stimulates cholangiocyte proliferation.
The above-mentioned studies give us reason to assume that the expression of serotonin in the glandular epithelial cells of GB and DC, DHC, DCH, and PDM in the pig marks cells similar to intestinal ECs whose role is probably related to the regulation of the function of the gland and the superficial biliary epithelium.
Mast cells are considered one of the serotonin sources in the gut, studied so far mainly in laboratory animals and humans (
30, 31). The present immunohistochemical study provides information for the first time on the presence and distribution of serotonin-positive mast cells in the pig’s GB, DC, DHC, DCH, and PDM.
Data on the ability of human mast cells to synthesize serotonin are controversial. Buhner & Schemann (
31) claimed that human mast cells in the mucosal layer of the intestine cannot synthesize 5-HT, contrary to the report of Yu et al. (
30). In all cases, activated mast cells synthesize and release 5-HT under pathological conditions in humans, contributing to hypersensitivity (
32). Individuals with mastocytosis who have low levels of circulating 5-HT are more likely to exhibit gastrointestinal and neurological symptoms (
33). The data on the presence and density of serotoninpositive mast cells in healthy porcine EHBD, PDM, and GB support the results of Yu et al. (
30) for a possible synthesis of serotonin by these cells.
The possible role of mast cell serotonin can be explained in different ways. 5-HT can influence the function of many types of immune cells. For example, 5-HT exerts chemotactic actions on mast cells and eosinophils (
34, 35). Dendritic cells (DCs) respond to 5-HT via the 5-HT3, 5-HT4, and 5-HT7 receptors to increase the expression of IL-6 (
36, 37). LPS-activated human monocytes are known to respond to 5-HT via 5-HT3, 5-HT4, and 5-HT7 receptors to increase secretion of IL-1β, IL-6, IL-8, IL12p40 and TNF-α (
38).
5-HT also inhibits monocyte apoptosis via the 5-HT1 and 5-HT7 receptors promoting inflammation (
39) with a crucial role in gastrointestinal inflammation (
40).
CONCLUSIONThe increased immunoexpression of serotonin in some of the secretory cells of the biliary glands and mast cells located in the propria, muscular, and serous layers of the
ductus hepaticus communis, ductus cysticus, the extramural part of the
ductus choledochus, the intramural part of the
ductus choledochus,
papilla duodeni major, and the gallbladder in the pig, suggests a possible synthesis of serotonin in these cells, which may participate in the regulation of physiological and pathological processes in these organs. We have assumed that serotonin, synthesized and secreted by ECs and mast cells regulates contractility (peristalsis), secretion, vasodilation, and pain sensation in the different segments of the EHBD, PDM, and GB most probably by activating 5-HT receptors. Future physiological and morphological studies are needed to confirm this role of serotonin.
CONFLICT OF INTERESTThe authors declare that they have no potential conflict of interest with respect to the authorship and/or publication of this article.
ACKNOWLEDGMENTSThis research was supported by Medical Faculty, Trakia University, Stara Zagora, Bulgaria, funded by Scientific Research Project No. 13/2017 on the topic “Investigation of the distribution of mast cells in the skin, carotid body and brain in rats, as well as in the extrahepatic bile ducts of the domestic pig in the norm” and by Bulgarian Ministry of Education and Science (MES) in the frames of Bulgarian National Recovery and Resilience Plan, Component “Innovative Bulgaria”, the Project No. BG-RRP-2.004-0006-C02 “Development of research and innovation at Trakia University in service of health and sustainable well-being”.
AUTHORS’ CONTRIBUTIONSIS participated in study conceptualization and design, data acquisition, immunohistochemical analysis and the provision of samples, performed the statistical analysis, interpreted the results, wrote the manuscript and approved the final version to be published.