Estimating the time of death after traumatic brain injury (TBI) in wildlife is a significant challenge in forensic veterinary medicine. The understanding of histopathological changes and predicting the survival time can prompt critical emergency measures and health management strategies for animals in managed care. Glial fibrillary acidic protein (GFAP) is a wellestablished astrocytic biomarker for diagnosing, monitoring, and predicting TBI outcomes. Moreover, the buildup of Beta- Amyloid Precursor Protein (βAPP) resulting from axonal damage is an energetic process intricately connected to the survival period following the injury. To date, no study has explored the accumulation of GFAP and βAPP in TBI chimpanzees. In human studies, the earliest reported time for detecting axonal injury postmortem in TBI using βAPP is approximately 30 minutes. This study aimed to investigate whether GFAP and βAPP staining can be used to detect postmortem axonal injury within 30 minutes in TBI chimpanzees. Cerebral and cerebellar tissues from a postmortem TBI chimpanzee and control samples were screened for immunopositivity for GFAP and βAPP in neurons using immunohistochemistry and immunofluorescence. The results suggested that neuronal immunopositivity for GFAP was likely a staining artifact, as negative controls also showed neuronal GFAP staining. However, it was not easy to assume the absence of post-traumatic neuronal GFAP. Conversely, the βAPP assay results indicated that axonal damage can be detected within 22 minutes after death, marking the fastest recorded time to date and aiding in diagnosing severe TBI with short survival times. In conclusion, we demonstrated that the axonal damage in captivated chimpanzee caused by severe and sudden concussion can be detected with βAPP staining within 22 minutes.
Numerous captive chimpanzees exhibit a range of aggressive and hazardous behaviors, including disputes over trivial matters, stereotypic movement disorder, conflicts within and out-group rivals, agonistic behavior (related to mates or food), feces painting, coprophagia, feces-throwing, urophagia, or self-injurious behavior (SIB). Some of these behaviors have been considered potential indicators of zoochosis, neuroses, systemic nervous disorders, and psychosis. Among these aggressive behaviors, there is a potential for physical conflicts and subsequent severe injuries (
1).
Measuring cerebral biomarkers is crucial for developing new wildlife forensics methods to estimate post-traumatic survival time and time since death, particularly in demographic studies or pathological investigations of epidemic diseases in a specific geographical area. To date, no wildlife forensic studies have examined Traumatic axonal injury (TAI)-related biomarkers within the brain tissues of wildlife species (
2, 3).
Glial fibrillary acidic protein (GFAP) is a protein, that significantly increasing within astrocytes after a TIA injury. Blood levels of GFAP in TIA are linked to incidence, severity, and extent of life-threatening intracranial pathology (
4).
Evidence also suggests that blood levels of GFAP could predict worsening disability in chimpanzees with progressive TA injuries. Higher serum GFAP levels may indicate clinical relapses of TIA (
5). This case report will focus on the possibility that TAIassociated neuronal GFAP positivity is a staining artifact rather than a pathological consequence of astrocyte damage after traumatically induced axonal injury (
6, 7).
Another diagnostic criterion for determining the timing of traumatic TBI involves evaluating the histopathological patterns and microscopic appearance of diffuse axonal injury (DAI) using various histological staining techniques, such as Hematoxylin and Eosin stain, Silver protein stains, and Beta-Amyloid Precursor Protein Staining (βAPP) (
8, 9). The intra-axonal restricted diffusion of the βAPP, combined with its accumulation in intraneuronal neurofibrillary tangles, is an active energy-dependent process that relies on cerebral circulation and is linked to the post-traumatic survival time of chimpanzees (
10, 11). Currently, the earliest recorded time for detecting axonal injury post-TBI using the IHC method is 35 minutes.
The expression of βAPP increases with the postinjury survival time for DAI, being more frequently detected after 55 minutes and reaching its peak intensity and highest frequency at 28 hours after TBI (
12). There is a direct correlation between the intensity of accumulated βAPP immunoreactivity and the survival period after the injury. However, the extent of accumulation cannot determine the timing of the pathological injury. In the field of wildlife forensic neuropathology, we have observed head injury in birds of prey, resulting in survival times of less than 40 minutes (
13). In other cases, involving red kangaroos and olive baboons, we have witnessed near-instantaneous and sudden deaths. This prompts the question: What is the minimum possible survival time after such injuries?
Recent evidence indicates that the survival time following acute axonal injury in TBI can be determined using the amyloid precursor protein accumulation IHC detecting technique. This finding is crucial in wildlife forensics for diagnosing TBI in animals that perish rapidly after head trauma, which can occur during the capture, handling, or physical encounters with wild animals. It also sheds light on the uncertainties in the pathobiology of TIA in wildlife animals (
14).
This case report aims to investigate whether some rationale for βAPP staining for traumatically induced axonal injury can be detected in the brain histopathological necropsy of wild animals following fatal injuries. By addressing this question, the report offers further insight into how quickly this IHC method can be demonstrated after fatal TBI and may provide a deeper understanding of the pathophysiology of TIA injury.
MATERIAL AND METHODSCase reportThe case report discusses the sudden death of a 15-year-old female chimpanzee at Tehran Zoo due to a fight, physical injury, and a blow to the head. It occurred during a dispute between a 31 year-old male chimpanzee weighing 75 kg and a 15-year-old female chimpanzee weighing 36 kg over food. In a sudden assault, the male chimp struck her with full force, resulting in immediate fatality. Despite intervention, the severity of the injuries led to unsuccessful attempts to save the chimpanzee, and she passed away 3 minutes later. Given the significance of the matter, the animal was promptly sent to the Necropsy Department, and the examination began 8-minutes post-mortem. The necessary brain samples were collected from different parts, exactly 22-minutes post-mortem.
Neuropathology examinationA standardized necropsy examination and sampling (unique documents number G117 and G097) were conducted according to established guidelines and protocols for animals with TBI in September 2023. (
15). During the necropsy examination, the brain was dissected by cutting into the coronal plane (frontal plane) at a 4 mm thickness. The slices were meticulously examined based on the topographical anatomy regions.
1. Subsequently, a complete set of tissue blocks was collected for histopathological and IHC studies, including six sections.
2. The cerebral cortex with subcortical white matter from the central semi-oval area next to the macroscopically verified contusions.
3. The cerebrum region is opposite to the pericontusional penumbra region, also known as the contralateral brain cortex (CLC).
4. The deep, polymorphic layer of the dentate gyrus of the hippocampal formation (CA4 region of the hippocampus (HC).
5. Cerebellum (little brain) (CB).
6. The frontal cortex contains four central gyri(FC).
7. Pericontusional Penumbra Region (PPR).
The obtained samples were divided into two parts, and a portion of those used for immunofluorescence was frozen on dry ice and stored at -80 °C.
Tissue preparationChimpanzee brain necropsy samples were fixed in 10% formalin for 42 hours. Formalin-fixed, paraffin-embedded tissue blocks (FFPE) were then prepared. The control blocks were collected from chimpanzees that had died in captivity in the past years from ailments associated with aging, with no acute or chronic injuries of the Central nervous system (CNS). First, routine Hematoxylin and Eosin (H&E) stains were performed to assess the morphological framework and to identify potential trauma-induced or inflammatory histological changes. Subsequently, IHC staining was conducted to detect the presence of two substances, βAPP and GFAP. Two IHC processes were carried out simultaneously, as follows:
Tissue processing and IHC stains were performed according to the Amyloid Precursor Protein Antibody Staining Protocol for IHC, as updated by Novocastra Labs. All chimpanzee brain sections were stained with βAPP using the BONDMAX Fully Automated IHC and ISH Staining System (Rankin Biomedical; Michigan, USA). The primary βAPP antibody (Anti-Amyloid antibody, ab12271, Abcam Biotechnology company) was diluted to 1/100. The sections were examined using high magnification X600 and X800 to assess the immunoreactivity of βAPP and accumulated deposits in the axons of white matter. Their shape, pattern, frequency, extent of distribution, and the average number of βAPP accumulated deposits per 10 × High Power field in each block were recorded. To investigate the immunopositivity for GFAP in neurons, the immunolabelled slices were incubated with 1 μg/ml of the primary antibody Monoclonal Purified anti-GFAP Antibody (BioLegend, Inc., USA) for 60 minutes at room temperature following antigen retrieval using Retrieve-All Antigen Unmasking System 3: Acidic, 100X (Catalogue No. 927601). GERPN1231-100UL (Streptavidin-HRP Conjugate-Cytiva RPN1231-100UL) was used for detection, followed by hematoxylin counterstaining, according to the provided protocol. All steps were performed by BONDMAX Fully Automated IHC and ISH Staining System (Rankin Biomedical; Michigan, USA). Twelve coherent “high-power field” (HPF) images were assessed for each brain region slide, resulting in a standardized investigation area of 3.42 mm2 per region. The commercially available object counting application Orbit Image Analysis (Orbit 2.52 with multichannel + Omero 5.3x support) (An opensource whole slide image analysis tool) was used to evaluate the slides in all twelve digital HPF images at 600 and 800×. Only CNS cells with distinct cellular immunostaining and differential protein expression, localization, and distribution at the CNS parenchyma, identified as neuronal in origin, were counted.
Nucleus morphological diversity, nucleolus location, distribution of nuclear chromatin patterns about the pathologic landscape, phenotype and connections of neurons, and location of neuron boarders were used as established morphological criteria to differentiate neurons from neuroglia (non-neuronal cells).
Immunofluorescence was performed to validate the results of immunohistochemical findings using neuronal phenotypic marker for ‘Neuronal Nuclei’ (NeuN) as a specific neuronal marker of chimpanzee brain tissue.
Statistical analysesData analysis was performed using GraphPad Prism 9.5.0.730 (GraphPad Street. Fl. 26Boston, MA 02110, USA) and Microsoft Excel version 365 update (KB5010321) (Microsoft). SPSS Kolmogorov-Smirnov and Shapiro-Wilk tests were utilized to assess the normal distribution of the data. Pearson Correlation Coefficient (r) and Spearman’s rank-order correlation were reported for parametric and non-parametric statistical data, respectively. The absolute number of GFAP immunopositivity in neurons was correlated with the total number of GFAP-positive astrocytes of the respective brain region and the modified immunoreactive score (IRS). P-values of 0.05 or less were considered statistically significant.
RESULTSNecropsy findingsThe necropsy revealed a nasal fracture, flail chest, hemothorax, internal eyelid rupture, corneal rupture, and orbital fracture. The lungs were heavily bloody and weighed down by the widespread infiltration of blood. However, other internal organs, such as the kidneys, spleen, liver, and digestive tract, remained undamaged. All internal organs were collected for histopathologic analysis. The skull gross findings (
Fig. 1) included only slight hemorrhage traces on the skin of the right parietal bone area, prompting a cut for additional examination. The continuation of the necropsy revealed a compound fracture (comminuted depressed fracture) at the right parietal bone (top of the posterior temporal line), along with a separate fracture of the right orbital roof. There was also a significant subdural and subarachnoid hemorrhaging, cerebral edema, and a mid-line shift towards the left side. Gross pathologic brain findings revealed contusion in the right frontal lobe with severe hemorrhage in the ventricles.
Histopathological findings in brain rissueThe brain’s histopathology (
Fig. 2) showed diffuse and basal subarachnoid hemorrhage. Contusions were visible in the temporal and frontal lobes, with the coup injury evident. Unilateral brain herniation and expanding infratentorial mass led to tonsillar herniation associated with hemorrhage and necrosis. Herniation due to hematoma formation and displacement by the skull fracture resulted in the flattening of gyri in one cerebral hemisphere, and the corpus callosum and fornix had small areas of hemorrhage, indicating microvascular injury. In the hemorrhagic brain areas, there was widespread neuronal pyknosis.
βAPP immunohistochemistry findingsAll samples tested positive for beta-amyloid precursor protein, with sequential accumulation observed in various topographical regions, including the cerebral cortex, corpus callosum, CLC, CA4 region of the HC, cerebellum (CB), FC, and PPR, with high frequency in certain regions (
Table 1) (
Fig. 3,
4,
5).
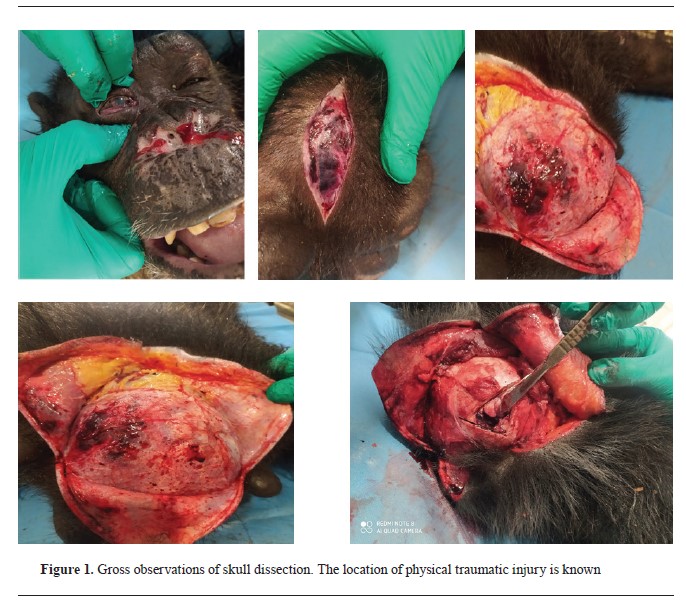
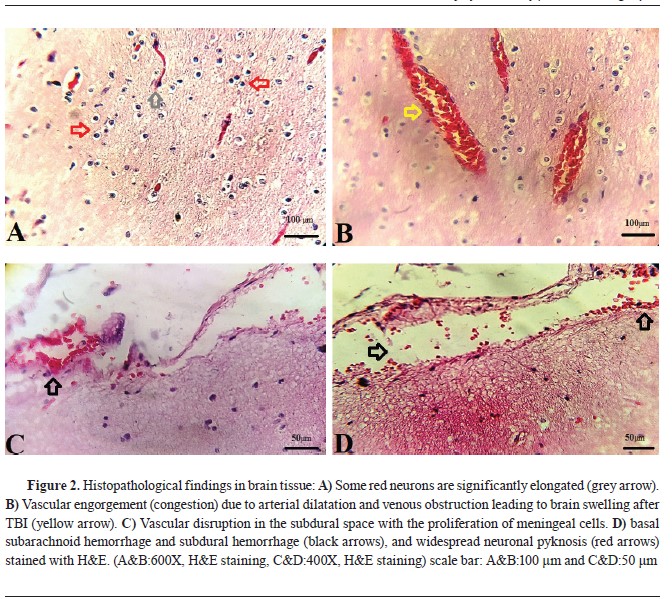
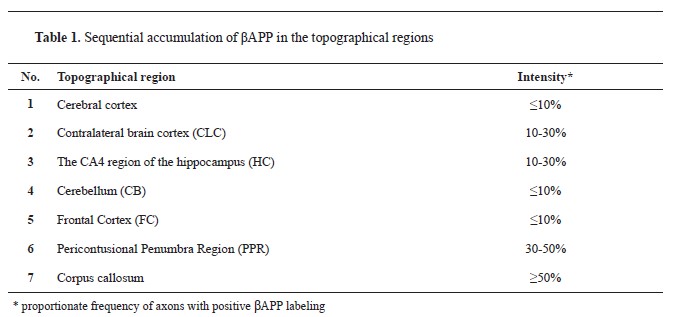
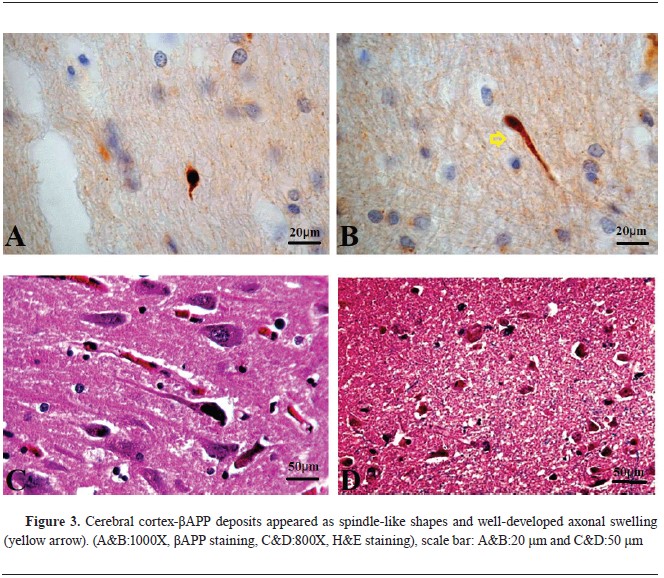
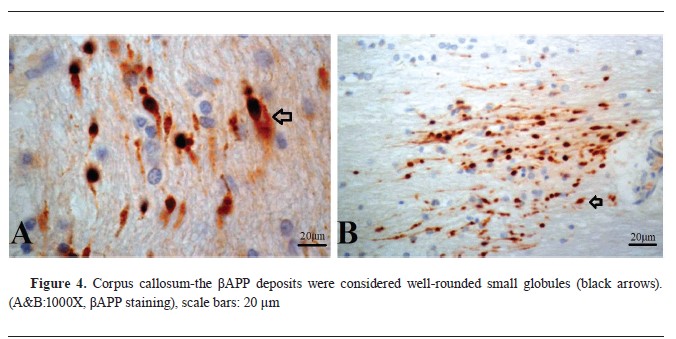
Astrocytic GFAP expression findingsThe investigation of GFAP immunopositivity in neurons in the six investigated chimpanzee brain regions (Cerebral cortex, CLC, HC, CB, FC, PPR) showed that TAI-associated neuronal positivity for GFAP is a staining artifact rather than a reactive process following traumatically induced axonal injury. Therefore, it has no diagnostic value in this study.
DISCUSSIONUnderstanding how captive chimpanzees die and the factors influencing their progression toward death are relevant to discussions on animal rights and welfare (
16). It is essential to comprehend the causes of death and their impact on the quality of captive chimpanzee life for welfare inspections. Two categories emerge when examining the most critical factors of chimpanzee mortality in captivity: internal and external factors, including traumatic ones. Heart diseases and, to a lesser extent, neoplastic causes are the primary internal factors leading to death. Regarding external factors, infectious diseases and accidental deaths are the most common causes (
17).
Thirty-two post-neonate necropsy reports listed various types of trauma as the cause of death, including those due to accidental injuries, attempts to escape from the zoo, and injuries sustained from conspecific aggression. For instance, five male chimpanzees escaped their primary enclosure in Sweden’s Furuvik Zoo, resulting in three deaths and one serious injury. In contrast, another chimpanzee was electrocuted at the Yagiyama Zoological Park. These tragic deaths which can be caused by human, engineering, or management failures may be preventable (
18).
In another report, two cases of drowning occurred when chimpanzees entered a water moat at the Detroit Zoo, but no such events have occurred at accredited zoos in the past 15 years. Fatal agonistic encounters are proportionately shared in wild chimpanzees, with violent encounters being the prevalent cause of death in some habitats (
19). Conspecific aggression has been reported as the most significant cause of death in male chimpanzees in their third to fourth decade of life (
20, 21, 22).
Injury-related deaths due to conspecific aggression remained relatively consistent over 30 years, with some occurring during daily life, social introductions, or violent conflicts between communities. While these deadly encounters are challenging for managers and keepers to be accepted, they are, in many ways, the result of efforts to expand, diversify, and improve the quality of social groupings for chimpanzees (
23).
The design and maintenance of larger social groups allow for enhanced social lives but also increase conflict. However, no proof supporting chimpanzees in smaller groupings would reduce such aggression and injuries. Additional research in this regard is infrequent but essential, as understanding lethal interactions in managed chimpanzee groups allows for progress in reducing the chance that they will occur.
Studying pathological injuries may be a proxy for fatal aggression risks. It is necessary to comprehend that aggression and injuries are not atypical in their society and that serious injuries represent only a tiny proportion of all managed chimpanzees (2.7%) (
23). However, since these severe injuries are rare, critical, and seriously life-threatening, they can cause irreparable harm. Therefore, the histopathological nature, time of occurrence, extent of injuries, and other factors significantly impact management in critical situations (
24).
The extent of injuries, the amount of damage caused, and the pillars of a standard pathology study about these injuries all trace back to the main factor: the time of occurrence of the pathological lesion and the period that has passed since the injury. Knowing the exact time of the accident allows for steps such as damage management and expected histopathological patterns to be considered. Therefore, the primary goal of this study is to determine the early time of histopathological damage to the central nervous system and its implications (
25, 26). For the first time, a study has been conducted regarding the status of two critical markers, GFAP and βAPP, in order to ascertain the rate of occurrence, time of occurrence, and their relationship with the occurrence and range of pathological damage in the chimpanzee’s central nervous system.
Previous work by Troakes in 2022 (
27) provided evidence that using βAPP immunohistochemistry, axonal damage can be detected within 30 minutes after severe head injury. This issue is challenging to discuss in forensic veterinary medicine, especially wildlife. In Troakes’ study, they estimated the time to death from prehospital emergency service information.
In contrast, in our study, we could immediately attend the bedside of the deceased chimpanzee and accurately measure the exact time of respiratory arrest and death after trauma by questioning the zookeeper. This chimpanzee is a case with injuries incompatible with the period of immediate survival, such as a severed head and complete severance of the spine.
Another significant contrast is that Troakes examined human cases, while our investigation focused on rapid death in a chimpanzee. Hence, a potential reason for detecting βAPP in shorter survival times could be that our study analyzed a distinct species with its unique nervous system, brain, and blood supply. The intensity of the force in this study and the gravity of acceleration and deceleration of brain tissue are anticipated to be more pronounced than the injuries documented in their study. This matter can be comprehended by delving into the pathophysiology of axon damage and the physiological role of βAPP, as well as by exploring the diverse forms of TBI and their varied impacts on different brain regions in other species, notably nonhuman primates like baboons and chimpanzees. It is now evident that TAI is prevalent across all TBI severities. Nevertheless, the extent of injuries varies among wildlife and species based on the force load and the level of brain acceleration and deceleration, which is directly linked to the physically altered axons (
28). In cases of mild-to-moderate traumatic brain injury, such as those seen in monkeys falling from moderate heights or in head injuries during male elephants’ musth period, there might be a mild and temporary transmembrane ionic disruption of axonal membranes, which is potentially reversible.
TAI is marked by increased squid axon membrane permeability to ions, mitochondrial swelling, involvement of mitochondrial permeability transition (MPT), and traumatic compaction of the axonal cytoskeleton (
29). Mild-to-moderate TAI may not lead to axonal injury or eventual transection. However, it could trigger retrograde axonal transport, which is considered as a potential mechanism for accumulating anterograde axonal transport products, such as Beta-Amyloid Precursor Protein (
30). On the other hand, severe traumatic brain injury results in direct primary Traumatically Induced Axotomy or significant disruption of neuronal cytoskeleton translocation, leading to secondary axotomy and induced apoptosis. Secondary axon degeneration and Distal Axonopathy following concussions are characterized by the emergence of Axon Retraction Balls and Varicose Veins within approximately 60 minutes post-injury, with disconnection occurring around 5 to 9 hours later. These changes can be visualized through βAPP immunoreactivity (
31).
In cases of severe traumatic brain injury, such as intense fights between large carnivores or physical conflicts between gorillas and chimpanzees, diffuse axonal injury and shearing of the axon’s fibers can result in complete disconnection through primary traumatically induced axotomy. However, very severe head injuries, like immediate decapitation in crocodile attacks on victims, hawk attacks on smaller birds, or the use of tools to crush heads in nonhuman primates, may also lead to diffuse axonal injury and rapid accumulation of transported products in the injured axons, including the βAPP. This accumulation can result in the appearance of small, well-defined bumps, as observed in our chimpanzee. These bumps likely indicate morphologically normal but functionally hindered axons with disrupted regular axoplasmic transport (
32).
CONCLUSIONIn the current study, we have demonstrated that the axonal damage in captivated chimpanzee caused by severe and sudden concussion can be detected with βAPP staining within 22 minutes. This result is an essential finding in the discussion of wildlife pathology and its legal aspects, aiding in diagnosing head injuries with very short survival times. One possible explanation for why we could detect βAPP accumulation earlier than previous studies is the speed of the procedure in examining the lesion, the method of identifying the time of death, and the time of survival after the injury.
CONFLICT OF INTERESTThe authors declare that they have no potential conflict of interest with respect to the authorship and/or publication of this article.
ACKNOWLEDGMENTSThe authors would like to express their appreciation to the Eram Zoo, Tehran, Iran for their collaboration, and support during all procedures of this experimental research.
AUTHORS’ CONTRIBUTIONSPM performed the measurements, PM and AB were involved in planning and supervising the work, PM and KA processed the experimental data, performed the analysis, drafted the manuscript and designed the figures. PM and RF performed the microscopic studies. PM and EE manufactured the slides and characterized them with microscope, PM performed the IHC methods. PM and SM aided in interpreting the results and worked on the manuscript. All authors discussed the results and commented on the manuscript.