Infectious laryngotracheitis (ILT) is a WOAH-listed respiratory disease in poultry caused by Gallid alphaherpesvirus 1, known as ILT virus (ILTV). We monitored two unvaccinated commercial layer flocks of 46- and 64-weeks old birds, more than 3 months after the onset of ILT. For this purpose, tracheal swabs, cloacal swabs, and blood samples were collected. Molecular and serology results were compared with the mortality data. The increased mortality in flocks 1 and 2 lasted 9 and 15 days, reaching 13.0% and 11.3%, respectively. We isolated the virus by inoculation on chicken embryo’s chorioallantoic membrane. Tracheal swabs were positive at each sampling point, but cloacal swabs were negative. Based on the molecular and phylogenetic analysis of the ICP4 gene, the ILTV closely matched vaccine strains. In flock 1, seroconversion was evident at the second sampling (day 15). Thereafter, an increase in antibody titer was observed, eventually achieving levels that were nearly identical to those on day 15 and on 109. During the acute period of the outbreak, seroconversion was already visible in flock 2, and a similar pattern was then seen as in flock 1. Three months after the outbreak, the virus DNA was still persistently detected in tracheal swabs.
ILTV causes acute respiratory disease, mainly in chickens, but also in other galliform birds such as peacocks, pheasants, turkeys, and guinea fowl (
2, 3). It is characterized by varying clinical appearances which can be manifested in mild or severe forms. The mild form is characterized by low morbidity and mortality, sinusitis, conjunctivitis, catarrhal tracheitis, nasal discharge, poor egg production, and poor weight gain. The severe form is associated with high morbidity and mortality, severe respiratory impairment, and expectoration of bloody mucous due to hemorrhagic tracheitis (
2, 3). Currently, there are no indications that the virus can be transmitted vertically. The horizontal route by indirect or direct contact is the most dominant way of infection. Indirect spreading can occur by contaminated fomites such as contaminated equipment, litter, feed bags, feathers, vehicles, dust, beetles, mealworms, footwear, clothes, movement of people, and wind (
4). The virus is infective at temperatures between 13 and 23 °C for 10 days up to 3 months in exudates from the respiratory tract and chicken carcasses. It can persist for 3 days at 11–19.5 °C in battery cage feces (
5). The ILTV enters the host predominantly via respiratory and ocular/conjunctival routes and to a lower extent via the oral route (
5). Different patterns of viral replication were observed in trachea and conjunctiva in chickens experimentally infected via different routes (
6). Once infected, chickens start to shed the virus before the beginning of the clinical signs (
7). Following the acute phase of infection, the virus is shed intermittently because of its maintained latency in the trigeminal ganglion and the trachea (
8, 9).
Outbreaks of ILT are occurring worldwide regardless of the use of live attenuated vaccines as a control tool (
10). However, endemicity was reported virtually in every country or region with poultry industry where live attenuated vaccines were widely used. Both field and vaccine strains are implicated as the source of the outbreaks as this can be easily confirmed by molecular methods on the gene or genome level (
10). Disease outbreak initiated by regained vaccine strain virulence has been reported in numerous studies and was recently reviewed in the context of new vaccination approaches (
11). Recently, recombinant strains were also designated as a cause of field outbreaks with the capability to replace the circulating genotypes and thus become the most prevalent strains (
12). Recombinant strains have been shown to be highly pathogenic in experimentally challenged birds (
13). Even live attenuated vaccines can produce recombinant progeny in experimental conditions when given subsequently in chickens (
14). Live chicken embryo origin (CEO) and tissue culture origin (TCO) vaccines were also implied in the recombination events in the field (
15). Antibody monitoring (e.g., ELISA or other techniques) is not useful to assess the level of protection against ILTV because the cellular immunity plays the crucial role in the immunological reaction (
16). Conversely, the ELISA technique has been mainly used for estimating the seroprevalence (
17) and the exposure to ILTV of non-vaccinated flocks. Initially, PCR-RFLP was used to discriminate between CEO, TCO, and wild-type strain vaccines by multiple target genes (
18). Recently, 14 genotypes of ILTV have been determined based on the allelic patterns of the six variable regions of different genes extracted from the complete genome sequence of 16 isolates (
19). Still, the sequencing and phylogenetic analysis of the two portions of the infected cell protein 4 gene (ICP4) proved to be a reliable method for the differentiation between a vaccine and a field-type strain (
20).
According to the surveyed literature, no studies describe the antibody pattern against ILTV during the acute phase of the disease and following 3 months after the recovery. Moreover, there is a scarcity of data studying the virus detection of convalescent birds in real-life scenarios/outbreaks, unlike in experimental settings. The current study aimed to assess the virus detection dynamics in tracheal and cloacal swabs, the seroconversion during and after ILT outbreak in commercial layers, clinical signs, gross lesions, and mortality rates in the ILT-affected commercial flocks. Additionally, the study aimed to detect and assess the effect of
Mycoplasma synoviae (MS),
Mycoplasma gallisepticum (MG), influenza A virus (AIV), and Newcastle disease virus (NDV) on the ILTV-infected flocks.
MATERIAL AND METHODSClinical caseThe affected farm consisted of three premises of which two were housed with ILT non-vaccinated commercial layers of different ages while the third premise was previously depopulated. Тhe two ILT flocks were housed in two premises sharing a common wall. The same workers, vehicles, feeding, and litter removal equipment was used for both flocks. Each flock had a mixed population of
Lohmann brown and
Lohmann white layers. Flock 1 consisted of 5,292 birds at the age of 46 weeks at the time of housing and were placed in enriched cages. Flock 2 consisted of 3,264 birds at the age of 64 weeks at the time of housing, and were placed in conventional cages. The mortality was first noticed in flock 1, and nine days later, in flock 2. Clinical signs in the affected birds were reminiscent of acute respiratory disease accompanied by prostration in the terminal phase of the disease. Necropsy was performed on the cadavers from both premises and the larynxes and tracheas were processed for virus isolation and molecular investigation. In flock 1, post-mortem findings were compared between the days of markedly elevated mortality (day 5) and the days of receded mortality (day 15). In flock 2, a necropsy was performed only during the markedly elevated mortality and acute phase of the disease (day 6). Live chickens from both flocks were sampled at several sampling time points until 62 and 80 weeks of age, respectively. Dry tracheal and cloacal swabs were collected for molecular analysis. Blood was sampled for serological analysis. Epidemiological inquiry related to this event was done by interviewing the owner and workers using a questionnaire. The questionnaire consisted of a list
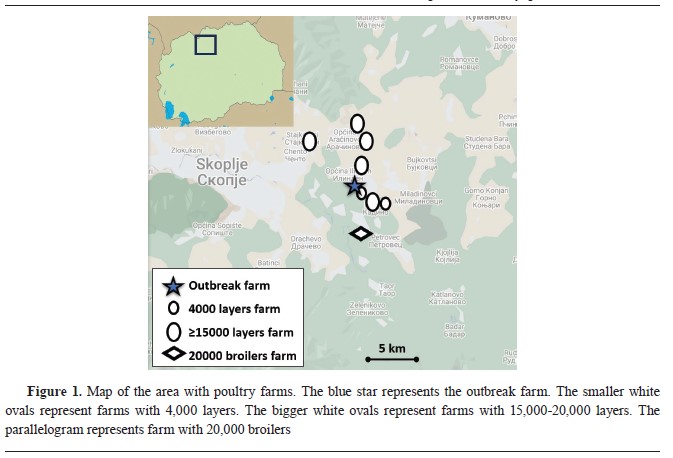
of questions related to the species, type of housing, category, number of birds present, number of sick birds, number of dead birds, date when first clinical signs were noticed, type of clinical signs, possible source of infection (animal husbandry practices, contact with wild birds, trade with live birds, trade with animal products, biosecurity practices, and records of visitors), and status of vaccination. The position of the farm in relation to other poultry farms in the area is shown in
Fig. 1.
Virus isolationNecropsy tissue samples were collected from flocks 1 and 2 on d5 and d6 of the outbreak, respectively. Larynx and trachea from both flocks were homogenized in an antibiotic medium, as prescribed by the WOAH, and 0.1 mL of the supernatant was inoculated on the chorioallantoic membrane (CAM) of nine-day-old embryonated chicken eggs. The inoculated embryos were incubated for five days at 37 °C. Subsequently, CAMs were macroscopically examined for the presence of typical plaques consisting of central necrosis and blurred perimeter. They were further processed for molecular identification (
21).
Serological analysisBlood samples from the brachial vein were aseptically taken in order to evaluate the seroconversion pattern. In flock 1, blood samples were taken during the acute phase of the outbreak when the mortality reached its plateau, i.e. d8 of the increased mortality, and then on d15, d22, d30, d36, and d109. In flock 2, blood samples were taken on d13 of the increased mortality and then on d21, d27, and d100. At every sampling, 10 blood samples were collected per flock which was sufficient to detect at least 1 positive sample with 95% confidence at an estimated prevalence of 30%. In total, 60 and 40 blood samples were collected during the study from flock 1 and flock 2, respectively. After the segregation of the serum from the whole blood, sera were stored at -20 °C until analysis. The sera were tested using a commercial ELISA test kit (CIVTEST
® AVI ILT, Hypra, Spain) according to the manufacturer’s recommendation.
Molecular and phylogenetic analysisTracheal and cloacal swabs were collected during the outbreak and in the convalescent phase. In flock 1, swabs were taken on d22, d30, d36, and d109 after the onset of increased mortality, and in flock 2 on d13, d21, d27, and d100. At the first sampling, 20 tracheal swabs were collected in each flock, while 10 tracheal and 10 cloacal swabs were collected on each subsequent sampling which was sufficient to detect at least 1 positive sample with 95% confidence at an estimated prevalence of 15% and 30%, respectively. Each pooled sample comprised of five individual swabs from the same flock, sampling date, and organ. Swabs were cut using clean scissors into 12 mL tubes containing 2 mL of sterile buffered phosphate saline. After vortexing for 1 minute, 200 μl of swab wash was taken and total viral nucleic acid was extracted using a High Pure Viral Nucleic Acid Kit (Roche Applied Science, Germany) following the manufacturer’s instructions. The obtained nucleic acid samples were stored at -80 °C until analysis and were tested in duplicates by real-time PCR for ILTV (
22), MG, and MS (
23) using a Brilliant III Ultra‐Fast QPCR Master Mix (Agilent Technologies, USA), Rotor‐Gene Q real‐time PCR cycler (Hilden, Germany), real-time RT-PCR for AIV (
24), NDV (
25) using a Brilliant III Ultra‐Fast QRT-PCR Master Mix (Agilent Technologies, USA), and the same PCR as for ILTV, MG, and MS.
The CAMs with plaques were frozen and homogenized in sterile buffered phosphate saline (1:1) and centrifuged at 1,000 x g for 20 min. Total DNA was extracted from the supernatant as described above. Using two sets of specific primers that bind at positions 181-204, 846-869 (ICP4-1), and 3,804-3,824, 4,418-4,440 (ICP4-2) of the ILTV ICP4 gene sequence that amplify 688 and 635 bp fragments, the DNA was tested using a conventional PCR method (GenBank accession number NC006623) (
17). The PCR was carried out using EmeraldAmp MAX PCR Master Mix (Takara Bio Inc, Japan) in Biometra T3000 PCR Cycler (Biometra, GmbH, Germany). Products that had been amplified were visualized on 1% agarose gel after electrophoresis. Sanger sequencing was performed in both directions on DNA samples obtained from excised gel fragments by Humanizing Genomics, Macrogen Inc. with the use of the same primers as for PCR. Following sequencing, the primer sequences were removed and the unprocessed nucleotide sequences were assembled. The BLASTn method was used to compare the obtained sequences with strains downloaded from GenBank to make identification and phylogenetic classification. In addition, nucleotide sequences from field ILT strains and CEO and TCO strains with low and high passage history were used for phylogenetic analysis. A maximum likelihood phylogenetic analysis was carried out, and MEGA7 software was used for the evolutionary studies (
26). GenBank accession numbers of the sequences in this study (GaHV-1/ chicken/Macedonia/ICP4-1/2019 and GaHV-1/ chicken/Macedonia/ICP4-2/2019) were OP805371 and OP805371, respectively.
Statistical analysisFor statistical analyses, we used STATISTICA software (version 8.0; StatSoft, Inc). To find differences in the mortality rate between the two flocks Chi-square test and the Fisher’s exact test were used. A non-parametric Mann-Whitney U test and descriptive statistics were performed on antibody titer values obtained from the two flocks. Significant differences were considered at P<0.05.
Ethical statementThe samples and data in this study were collected on a non-experimental farm practice registered at the Food and Veterinary Agency as part of the compulsory animal samples and data collection in case of an outbreak stipulated in the Veterinary Law (Off. Gazette of RM, 123/2015). During the sample collection, the birds’ tracheal and cloacal swabs and blood samples were collected by expert veterinarians under ethical standards and animal welfare requirements. All procedures in this study complied with the national Law for the Protection and Welfare of Animals (Off. Gazette of RM, 149/2014), which is harmonized with the Directive of the European Council on the protection of animals used for scientific purposes (2010/63/EU).
RESULTSClinical and post-mortem findingsBoth affected flocks of different ages showed the same variety of clinical signs including hemorrhagic conjunctivitis, serous discharge from the nostrils, severe respiratory distress, ruffled feathers, drop in egg production, in the terminal phase of the disease inability to stand and walk, sitting in the recumbent position, and diarrhoea. The dynamics and the extent of the mortality differed in both flocks especially at d2, d3, d4, and d9 when a statistically significant difference (p<0.001) was observed (
Fig. 2). The duration of the increased mortality in flock 1 was nine days totalling 13.0%, while in flock 2 was 15 days totalling 11.3%. Again, the difference in total mortality of the two flocks was statistically significant (p=0.023). However, flock 1 showed a higher peak in mortality than flock 2. Moreover, mortality rates were observed to rise in flock 2 nine days later than in flock 1. The drop in egg production was 21.5% and 20.4% in flock 1 and flock 2, respectively.
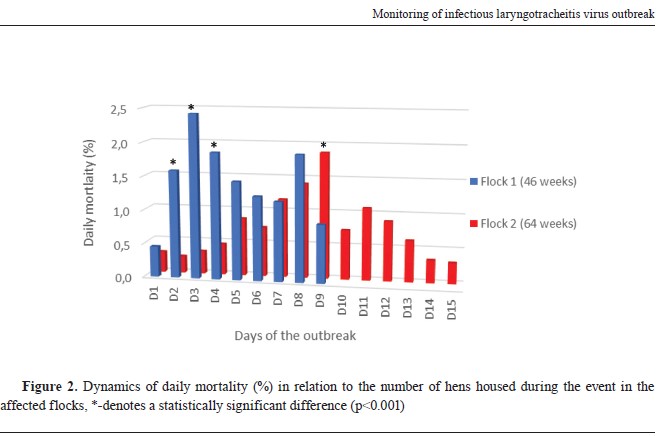
The post-mortem findings of the cadavers collected from the different phases of the disease differed in severity. Macroscopic lesions observed at the time of the acute phase of the disease, i.e., markedly elevated mortality (d5) were hyperemia of the conjunctivae, caseous plugs in the trachea, severe hemorrhagic tracheitis, pneumonia, and hemorrhagic infiltration of the ovaries. Conversely, when the mortality was almost back to normal, i.e., at the tail of the outbreak (d15) macroscopic lesions observed in flock 1 were catarrhal tracheitis, sporadic mild pneumonia, moderate hemorrhagic infiltration of the ovaries, and findings of secondary bacterial infection in the like of fibrinous pericarditis. Macroscopic lesions in flock 2 collected during the acute phase of the outbreak (d6) were identical to the lesions observed in flock 1 at d5.
Virus isolationThe virus was successfully isolated on the chicken embryo CAMs from the laryngeal and tracheal homogenates collected in the acute phase of the outbreak on d5 and d6 from flock 1 and flock 2, respectively. CAMs had typical macroscopic lesions of ILTV. Furthermore, CAM homogenates were positive when tested using real-time PCR.
SerologySeroconversion was visible in flock 1 at the second sampling (d15), with the highest antibody titer average and geometric meantiter. Following this period, the antibody level decreased, but a subsequent increase on d109 reached almost the same levels as on d15 (
Fig. 3). In flock 2 there were two samplings less than in flock 1. The first two samplings at d13 and d22 were with the highest antibody titers. Following this period, the titer decreased and at d100 there was a slight increase which never reached the initial titer level as was the case in flock 1 (
Fig. 3). No statistically significant difference was found at all sampling points between antibody titers of flock 1 and 2.
Molecular and phylogenetic resultsTracheal swabs were positive at every sampling point, but cloacal swabs were negative. They ranged from Ct of 28.8 and 29.8 to 24.7 and 26.5 in flocks 1 and 2, respectively (
Table 1). Additionally, tracheal and cloacal swabs were tested for MG, MS, AIV, and NDV. Tracheal swabs were positive for MS, cloacal swabs were negative, and all were negativefor MG, AIV, and NDV.
The phylogenetic trees based on the two ICP4 gene fragments of the Macedonian ILT strain isolated in this outbreak have shown different topologies (
Fig. 4). Nucleotide sequences of the two ICP4 gene fragments of the Macedonian ILT strain shared 100% identity with a range of vaccinal CEO strains as well as with CEO strains of low and high passage history in either tree. However, there are fewer strains that tightly cluster with the Macedonian ILT strain in both trees. These exceptions are Nobilis Laringovac (KP677881), Poulvac (KP677882), and live attenuated Serva vaccine (HQ630064) which share 100% identity only in a single tree. Nevertheless, they are closely positioned to the Macedonian ILT strain in the other tree sharing 99.83%, 99.84%, and 99.83%, respectively. On the other hand, Korean field ILT strain 30678/14/Ko (MH937565) is highly divergent in the first tree and identical to the Macedonian ILT strain in the second tree. Inversely, the Chinese virulent strain WG (JX458823) is identical in the first tree to the Macedonian ILT strain but forms a separate branch together with the Chinese vaccine strain K317 (JX458824).
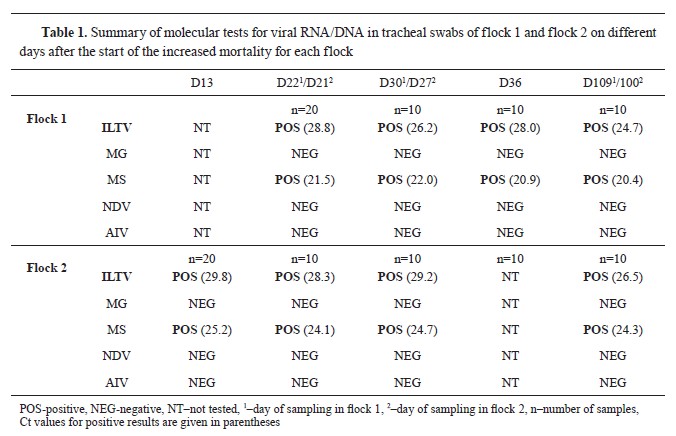
DISCUSSIONDespite the implementation of numerous ILT control strategies, ILT has persisted throughout the globe and expanded into new regions, resulting in significant economic losses for the poultry industry (
5, 27). Outbreaks of this disease have been recently reported in the region including five layer farms and a breeder farm in Serbia (
28), an organic broiler farm and surrounding flocks in Greece (
29), and commercial and backyard poultry flocks in Slovenia (
30). In all these field outbreaks, farms with different housing systems (cage, floor, extensive, intensive) were affected. Like in the current study, these studies did not report the correlation between the housing system and the severity of clinical signs, morbidity, mortality, and transmission potential although this not beeing an iam of the studies. . Despite that most outbreaks in the field are brought on by viruses that are identical to CEO vaccine strains (
4), wild strains of ILTV were also involved thus raising concern about its spread within the countries of the region. Although, both mild and severe forms of ILTV can be distinguished based on the clinical appearance of the disease (
2, 3), in our case, it was difficult to ascertain the clinical form because the range of clinical signs and post-mortem findings could be attributed to both forms of the disease. Specifically, the acute phase of the disease was accompanied by high mortality with clinical and post-mortem findings resembling to the severe form. In the later phase when mortality declined, clinical and postmortem findings resembled a mild form of the disease which could also be attributed to the gradual increase of the flock immunity as the outbreak went on. Histopathology could support the clinical findings but it was not in the scope of this study. The mortality pattern was different in both flocks and was statistically different at d2, d3, d4, and d9 (p<0.001). Flock 1 which was younger, had shorterlasting but a sharper increase in mortality. Flock 2 had an opposite pattern of mortality, lasting six days longer, but never reaching the maximum as in flock 1. Moreover, the total mortality was significantly higher in flock 1 (13.0%) than in flock 2 (11.3%) (p<0.023). The positive correlation between high mortality and age was evident in the mixed-age poultry flocks where younger birds showed more severe clinical signs and higher mortality than older birds (
30). The dynamics of the viral introduction and replication could have been more extensive in flock 1 in which had probably higher initial exposure to the virus. Maybe, smaller number of birds were initially exposed in flock 2 resulting in lower and slower rate of infection spreading as well as a longer duration of the disease. Comparing the clinical scores of 35 and 74-week-old challenged birds, it appears that clinical signs are less visible with higher age although the viral load is not affected (
27). The interval between the start of the disease in flock 1 and flock 2 was nine days and fits well within the field outbreaks incubation period of 6 to 14 days (
3). This indicates that it was very likely that the infection spread from flock 1 to flock 2. The primary source of the virus introduction was not clear. The epidemiological inquiry revealed that 14 days before the start of the outbreak а truck with dirty crates visited the farm to transport the spent hens to the slaughterhouse. The same truck was regularly servicing the poultry farms. Although it cannot be concluded with certainty that the virus was introduced by the dirty crates, the time of the visit of the truck corresponds to the ILT incubation period. ILT is not a notifiable disease in Macedonia thus it is possible that the ILT was already present on another farm which served as a source of the infection for the studied flocks. Other authors also have speculated about the plausibility of this scenario (
28). Interestingly, one month later, ILTV was diagnosed by real-time PCR in another farm at 1.45 km distance, raising concerns that it was circulating in this area (
Fig. 1).
Detection of antibodies using ELISA can be used as a tool to perform seroprevalence studies or to detect field outbreaks (
16, 31, 32). However, the determination of the quantity of ILT antibodies is not indicative of the level of protective immunity (
32). There are studies examining the vaccineinduced titer (
33) and post-challenge titer (
34), but according to our knowledge, no study has monitored the level of antibodies during threemonth post-outbreak period. To expand the knowledge about the persistence of ILT antibodies, we tested the subject chickens three months after the outbreak and we found that there was still a presence of circulating antibodies in the sera which could be correlated to the permanent detection of the viral DNA from the tracheal swabs. In another study, a carrier state of the ILTV was demonstrated in experimentally infected and recovered birds up to eight-week post-challenge period (
8). In our study, we detected ILTV DNA in tracheal swabs three months after the field infection. It is known that common stressors can reactivate latent ILTV infection (
35) which could be the case in our study. Despite the ILTV-positive finding at the later stage of the infection, there was no increased mortality or drop in egg production probably due to the acquired immunity. The reactivation ability of ILTV was first demonstrated in live vaccine viruses (
36). In experimentally infected chickens with ILTV field strains, positive detection was observed in multiple organs and entire segments of the intestinal tract as early as 28-days post-infection which could suggest on systemic replication (
37, 38). The colonization of the ILTV of the intestines could lead to the shedding of the virus via the cloaca. However, in our study, we could not detect the virus from the cloacal swabs of clinically recovered birds in none of the sampling points. This could be explained with virus absence in the intestines after recovery or due to the different tissue tropism. Cloacal swabs were of limited or no value for the diagnosis or ILTV infection screening in this study.
In order to infer phylogenetic relationships, we sequenced two fragments of the ICP4 gene using a validated test to distinguish between vaccine and wild-type viruses (
20). From the phylogenetic trees with different topologies, the Macedonian ILT strain clusters with several CEO ILT vaccines in both trees. The strain in this study had the highest nucleotide identity with the CEO vaccine (EU104900) across the two fragments of the ICP4 gene. To demonstrate eventual recombination, whole genome sequencing should be performed. Whole genome studies of the ancestral and current ILT strains revealed that there is a high genetic similarity of the ILTVs over time and that the current epizootics are caused by virulent vaccine-type viruses (
10, 39). Therefore, the results of this study corroborate an urgent need for greater emphasis on research concerning safety issues of the ILT vaccine (
40) since the vaccination can easily produce a serious counterproductive effect in non-vaccinated birds. Efficient but safe ILT vaccines are without no doubt essential for disease control since cleaning and disinfection programs might be insufficient (
11).
Co-infections are very common with ILTV infection involving viral and bacterial pathogens and can occur in farm and backyard settings (
30). In our study, both flocks were co-infected with MS which could be related with fibrinous pericarditis found in the later stage of the disease. Furthermore, all tracheal swabs collected during the observation period were MS-positive pointing to chronic co-infection. It is difficult to quantify how co-infections contribute to the severity of the clinical signs, morbidity, mortality, and duration of the disease in the field. It can be postulated that ILTV was largely responsible for the clinical manifestation in the birds because they were concomitantly infected with ILTV and MS, and the clinical signs and mortality appeared only during the acute disease. A recent study revealed that MS was detected concomitantly with all ILTV infections (
30). However, this study was performed at one time point, unlike our study.
CONCLUSIONAlthough outbreaks of ILT occurred in the past, this study is the first report of ILT disease in Macedonia. The outbreak in non-vaccinated commercial layers was possibly caused by a CEO vaccine like virus, but this assumption requires comprehensive genetic studies which are not commonly available. This calls for the development of a simple and reliable test for discrimination of field from CEO vaccine-like ILTV strains. Even three months after the beginning of the outbreak, the antibody titers did not show a waning pattern in either of the two observed flocks. The virus DNA was persistently detected in tracheal swabs although mortality receded after 9 and 15 days, respectively. ILTV DNA was not detected in cloacal swabs during and three months after the outbreak onset.
CONFLICT OF INTERESTSThe authors declare that they have no known conflict of interest in conducting the current study.
ACKNOWLEDGMENTSThe authors would like to acknowledge the laboratory staff at the Faculty of Veterinary Medicine–Skopje and Poultry Centre of the Croatian Veterinary Institute. Also, the authors appreciate the cooperation of the staff at the farm subject of this study.
AUTHORS CONTRIBUTIONAD wrote the original draft. VS made the data curation. Both authors contributed equally to the conceptualization, design, methodology, writing, and editing of the manuscript.