Canine Distemper Virus (CDV) infection causes a multifocal demyelinating progressive disease within the central nervous system (CNS) that results in wide range of neurological symptoms. Pathological changes in the brain or CNS could be observed by analyzing cerebrospinal fluid (CSF). Therefore, this study aimed to investigate the concentrations of amino acids in the CSF of dogs with neurological form of CDV infection, and to identify amino acids as markers that can be used in the diagnosis, pathogenesis, and treatment of the disease. Heathy dogs (n=6), confirmed by clinical and laboratory examinations (Healthy group), and CDV-infected dogs (n=10) with neurological symptoms, confirmed by clinical, laboratory, and rapid diagnostic test kits (Distemper group), were used. CSF samples were obtained with an appropriate method and were subjected to 1H-NMR analysis. Identification was made on 10, whereas quantification on 8 amino acids. L-tyrosine, L-phenylalanine, L-threonine, and L-alanine concentrations were significantly lower, while L-histidine and L-tryptophan were significantly higher than the Healthy group (p<0.05). It was concluded that L-tyrosine could be used for assessing mental status changes, L-phenylalanine for evaluating neuroprotective responses, L-threonine and L-histidine for gauging the extent of neurodegeneration and ventricular degeneration, L-alanine for exploring cellular stress and energy metabolism, and L-tryptophan for understanding the process of sympathetic nervous system activation.
Metabolomics, which involves the thorough examination of small-molecule metabolites in a tissue of biofluids, provides valuable insights into the biochemical processes of a body system, compartment or organ under specific physiological conditions. It holds various applications for enhancing clinical diagnosis and treatment of CNS-related diseases (
6). CSF is a by-product of membrane secretion and plasma filtration. Its composition differs from that of the blood plasma, it reflects the cerebral metabolic rate, and is used in the transportation of waste products and nutrients from the metabolic processes of neurons (
7). Several authors have noted that the neurological form of CDV in dogs, with or without systemic involvement, can be detected and monitored by CSF samples which demonstrate greater sensitivity compared to mucosal swabs or whole blood samples (
4). Also, the potential relationships between neurodegenerative diseases, including CDV (
8), and amino acid concentrations have been emphasized in many studies, and it has been stated that the underlying mechanisms need to be elucidated (
9).
It was previously reported that changes in amino acid levels can be important prognostic factors in many diseases. Determining the amino acid profile in several conditions, including viral diseases, is important in terms of both diagnosis and supplementation of the individuals. Certain clinical trials have also demonstrated that substituting the lost amino acids in major disorders could lead to clinical healing (
10). The current study aimed to assess the relationship between CSF amino acid concentration in dogs with neurological form of CDV and the disease progression and prognosis, and to identify which can be used as markers for its diagnosis.
MATERIAL AND METHODSThe study protocol was approved by the Harran University Ethics Committee (session and committee approval number: 2021-010/326679 dated 16.02.2021). Also, the owner’s consent was obtained before the clinical evaluation and sample collection.
AnimalsThe samples were obtained from 16 dogs. The Distemper group included 10 dogs (4 males, 6 females) aged between 2-6 months, representing medium to large breeds such as Boxer, Anatolian shepherd, and mixed breeds. These dogs were presented for diagnosis or treatment due to symptoms indicative of neurological CDV infection, including fever, nasal/ocular discharge, cough, dyspnea, and as neurological findings like head tilt, ataxia, convulsion, nystagmus, and myoclonus (
4). The Healthy group included 6 dogs (4 males, 2 females), aged between 2-6 months, similar breeds, brought in for vaccination and/or routine check-ups.
Physical examinationsBody temperature, respiratory and heart rates measurements, as well as heart and lung auscultation, and palpable lymph nodes palpation was conducted. Additionally, otoscopic and fundic examinations were undertaken to exclude any diseases that might manifest with head tilt, nystagmus, and ataxia. A body temperature exceeding 39.2 °C and a capillary refill time shorter than 3 s (hyperdynamic state due to pain, fever, and/or infection), were considered as significant clinical indicators for the disease (
1, 4). The same set of examinations was performed in the Healthy group which was expected to have reference range values.
Blood samplingCephalic vein blood samples (5–10 mL) were collected from all dogs by using a minimal restraining approach to minimize stress. Blood samples for the complete blood count (CBC) were collected in tubes containing ethylenediaminetetraacetic acid (K3EDTA). CBC analysis was conducted using an autoanalyzer (Sysmex pocH-100i Automated Hematology Analyzer, Japan). The obtained CBC results were assessed based on the inclusion/ exclusion criteria and were not incorporated into the study.
Rapid diagnostic test applications and inclusion/exclusion criteriaAdenovirus 2, Canine Coronavirus, Canine Influenza virus, (Asan Easy Test CAV2/CCV/CIV Ag®, ASAN Pharm. Co., Ltd. Gyeonggi-do Korea, relative sensitivity: 93.10%, relative specificity: 97.50%), and Canine Parvovirus antigen tests (Asan Easy Test CPV Ag®, ASAN Pharm. Co., Ltd. Gyeonggi-do Korea, relative sensitivity: 97.96%, relative specificity: 97.50%) were conducted on the dogs in the Distemper group in order to rule out any concurrent diseases. Both ocular and nasopharyngeal secretions were collected using sterile wet swabs in order to confirm the suspicion of CDV infection. The CDV Ag test (Asan Easy Test CDV Ag®, ASAN Pharm. Co., Ltd., Gyeonggi-do Korea, relative sensitivity: 97.96%, relative specificity: 97.50%) was then conducted in accordance with the manufacturer’s instructions. Dogs with positive nasal/ocular CDV Ag test results were included in the Distemper group (n=10). Dogs that tested negative for CDV, CAV2, CIV, CCV, and CPV Ag, and with normal clinical findings, were deemed healthy and included in the Healthy group. Dogs lacking neurological symptoms but with positive CDV Ag test results, were excluded from the research.
Collection of cerebrospinal fluid samplesDogs from both groups were sedated via intramuscular injection with xylazine (Xylazin Bio 2%, Bioveta) in a dose of 1 mg/kg following blood collection, prior to the CSF tap. CSF samples were taken (1–2 mL) between the occipital and atlas bones, by using a 22-gauge, 1.5-inch stylet spinal needle (
11). In order to prevent obstruction of the airway, excessive bending of the head was avoided. No problems were noted during or after the procedure.
Preparation of samples and NMR analysisCSF samples were refrigerated and analyzed within 48 hours of sampling to maintain the biological pH and stability of amino acids. Prior to NMR analysis, the CSF samples were centrifuged for 10 minutes at 7,000 rpm after being equilibrated to room temperature for approximately 30 minutes. Sample preparation involved adding 20 μL of phosphate buffer solution (pH 7.5) prepared in 580 μL of dimethyl sulfoxide-d₆ (DMSO-d₆, CD₃SOCD₃) to a 2 mL Eppendorf tube containing a 60 μL aliquot of CSF. The phosphate buffer solution was prepared in DMSO-d₆ to ensure compatibility with the solvent system and to minimize interference in the 1H-NMR spectrum. Additionally, 5 mg of sodium 3-(trimethylsilyl)-[2,2,3,3-d₄]-1-propionate (TSP), an internal standard, and sodium azide as a preservative, were added to the mixture to achieve a TSP concentration of approximately 3 mM. The pH of the final sample was adjusted to 7.0 as needed. For subsequent analysis, the entire sample volume (600 μL) was transferred into a 5-mm NMR tube.
The 1H-NMR spectra were obtained at 26.5 °C using an Agilent 400 MHz spectrometer operating at 400.13 MHz, equipped with a 5 mm inverse detection probe featuring gradients on the z-axis. Samples were run in 5 mm Wilmad 507 NMR tubes. The spectra were recorded utilizing the Nuclear Overhauser Effect Spectroscopy (NOESY) presaturation pulse sequence with parameters set at 32 scans, a 30 s relaxation delay, 4 s acquisition time, 8223 Hz spectral window, and collection of 64 K data points, offering a digital resolution of 0.12 Hz. Post-acquisition Free Induction Decay (FID) processing involved an exponential line broadening factor of 0.3 Hz. Chemical shifts are reported as δ values (ppm), referenced to TSP (0.0 ppm) as an internal standard. The Agilent SpectrAA software was employed for acquisition and processing. The NMR protocol allowed for full signal relaxation over a 37 s overall relaxation delay. Therefore, utilizing a 0.3 Hz line broadening and 37 s relaxation delay enables the use of signal intensities for quantitation purposes. In crowded NMR spectra, employing signal intensities, rather than integrals, reduces errors induced by partial signal superposition. 600 μL of each sample (the standard 5 mm NMR filling volume) was used for a single NMR study after the collected CSF sample was reduced to the bare minimum volume required. Instead of taking two measurements with half the filling volume, one measurement with the regular filling volume produces better results because of improved shimming (higher signal-to-noise ratio) and increased sensitivity (better magnetic field homogeneity, which results in sharper lines in the spectrum). All NMR sample preparations and tests were performed by a single professional operator in order to reduce operator error. When the same operator was tested, analytical error was significantly lower than biological variation. For four different NMR samples prepared from the same original batch, analytical error for various amino acids ranged between 1-5%, while biological variation (expressed as % RSD) fell within the range of 10-45% (
12).
Identification and quantification of CSF amino acidsThe identification and quantification of CSF amino acids derived from raw 1D NMR spectra-FID files obtained using the Agilent device, was performed by employing the BAYESIL software. BAYESIL performs fully automated spectral processing and spectral profiling for 1D and 1H-NMR spectra obtained at different frequencies using standard instruments. During the spectral deconvolution process, BAYESIL divides the spectrum into small blocks and uses a probabilistic graphical model to show the sparse dependencies between these blocks. It then does approximate inference over this model to serve as a stand-in for spectral profiling, resulting in the most probable amino acid profile. BAYESIL, utilized for identification and quantification, encompasses a spectrum of spectral processing functions. It performs many procedures, including zerofilling, phasing, baseline correction, smoothing, chemical shift referencing, and reference deconvolution, starting from the raw spectrum. The quantified amino acids were computed as relative concentrations of the total area. Statistical calculations for the relative concentrations of amino acids were performed using BAYESIL (
13). NMR spectra visualization, simulation, and presentation were performed using a software package (MestReNova, MestreLab Research, Spain) and presented in
Fig. 1 and
2.
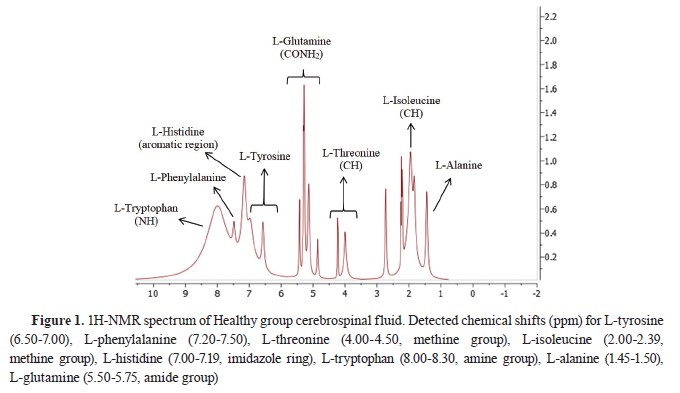
Statistical analysisThe statistical software SPSS 25.00 (SPSS for Windows®) was utilized for data analysis. A one-sample Kolmogorov-Smirnov test was conducted to determine whether the data followed a parametric or non-parametric distribution. The data, which were determined to be non-parametric, were evaluated as median (min-max) using Mann-Whitney U and Kruskal-Wallis tests. Statistical significance was regarded when p<0.05.
RESULTSAnimalsAnamnestic data showed that all of the dogs in the present study were given standard commercial dry dog food and had not received any vaccination or dewormer. The anamnestic data for the dogs in the Distemper group indicated that they had symptoms for the last 4 (2-8) days before admission, and that no treatment had been received. The physical examination findings are presented in
Table 1.
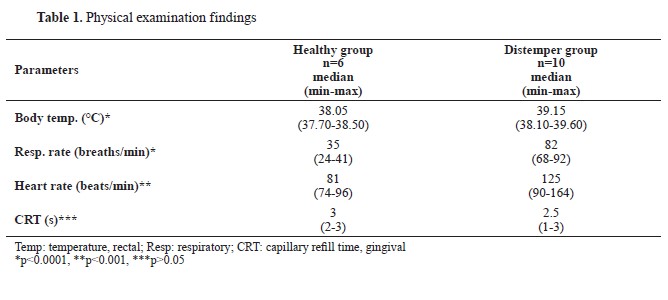
1H-NMR analysis of CSF samplesTen amino acid concentrations were determined in the CSF samples of the diseased and the healthy dogs. L-methionine and L-leucine concentrations could not be measured. L-tyrosine, L- phenylalanine, L-threonine, and L-alanine concentrations were significantly lower, whereas L-histidine and L-tryptophan concentrations were significantly higher compared to the Healthy group. L- isoleucine and L-glutamine concentrations were non-significantly different. The identified and quantified amino acids are presented in
Table 2.
DISCUSSIONIn the present study, amino acid concentrations of CSF samples of the dogs suffering from the neurological form of CDV infection were investigated by 1H-NMR approach. Compared to the healthy dogs, L-tyrosine, L-phenylalanine, L-threonine, and L-alanine concentrations were lower, and L-histidine and L-tryptophan concentrations were higher in the CSF samples of dogs with neurological CDV. Potential clinical significance was found for the following amino acids: L-tyrosine for assessing mental status changes, L-phenylalanine for evaluating neuroprotective responses, L-threonine and L-histidine for gauging the extent of neurodegeneration and ventricular degeneration, L-alanine for exploring cellular stress and energy metabolism, and L-tryptophan for understanding the process of sympathetic nervous system activation.
As a multi-cell pathogen, CDV can infect three distinct types of host cells: lymphoid, neurological, and epithelial cells. The generation of DL during the chronic phase of CDV infection has been previously studied. Severe non-suppurative leptomeningitis with multifocal demyelination and hemorrhages, as well as encephalitis with gliosis were reported (
14, 15). Important processes in DL include the decline in viral titers, changes in astrocyte maturation and plasticity, primary axonopathy, and possible Schwann cell-mediated regeneration. Certain CDV strains, such as the A75/17 strain, exhibit persistent virus infection in the CNS when they can invade certain brain regions without inducing an inflammatory reaction (
1). Certain CDV host cell receptors, such as the SLAM, which is expressed on activated T- and B-lymphocytes, dendritic cells (DCs), and macrophages, have been extensively researched. CDV is thought to be a systemic infection that affects a wide range of organs and tissues. These function similarly to the normal morbillivirus entrance receptors. Other extensively studied receptors include nectin-4, which is recognized as an epithelial cell receptor and is currently considered as a host-exit receptor (
16). This complex pathogenesis is responsible for the neural findings such as head tilt, nystagmus, circling, convulsions, complete or partial paralysis, behavioral changes, and dementia along with involuntary muscle contractions, twitching, and gum chewing (
17). Many common diseases exhibit significant heterogeneity, with varying phenotypes, distinct genetic mechanisms, and diverse responses to identical treatments. Consequently, the identification and investigation of biomarkers play a pivotal role in obtaining valuable insights for the diagnosis, prognosis, and management of these diseases (
18). An in-depth understanding of the current status of systemic metabolism is made possible by the omics based methodology known as “metabolomics,” which produces substantial information on metabolism. Studies involving dogs have seen an increase in the use of metabolomics. The field of metabolomics has enormous promise for clinical diagnostics. It is a useful tool for highlighting the changes in metabolism linked to the start and course of diseases, early disease detection, and customized treatment protocols (
19). Preventive and supportive care cannot be given until organ failure has already manifested. The simultaneous metabolic events occurring during multiple diseases may render similar laboratory findings which renders the diagnosis and interpretation cumbersome (
20). Identifying suitable biomarkers holds the potential to address several challenges, i.e. more precise differential diagnosis, understanding the onset and progression of diseases, assessing the effects of therapy, and establishing the timing of disease development. Differential diagnosis can be challenging, particularly in the early phases of neurodegenerative disorders which may result from diverse diseases. This may contribute to negative or inconclusive outcomes in the clinical trials. A reliable diagnostic biomarker can increase the treatment success, lower the expenses, and save the time. Individual therapy approach for each specific patient may additionally aid the treatment (
21). Analysis of the metabolites found in CSF can shed light on the metabolic changes associated with CNS diseases. These metabolites can be intermediate and final products of energy metabolism, neurotransmission, inflammation, and oxidative stress responses (
22). Age, diet, environment, breeding, and genetics were thought to be the main determinants of CSF metabolite concentration (
23). Considering that the animals included in the present study were of similar age, breed, and had similar diet, it can be remarked that the differences observed in the CSF samples of the diseased and the healthy dogs were not affected by these factors.
Tyrosine serves as the precursor for catecholamines, undergoing conversion to dopamine through the intermediaries L-dopa and the enzymes tyrosine hydroxylase and aromatic l-amino acid decarboxylase. Additionally, it is converted to noradrenaline by dopamine β-hydroxylase. Studies in rodents have demonstrated that orally administered tyrosine can effectively reach the brain. Tyrosine administration increases dopamine metabolites in CSF, like homovanillic acid, in rats and in patients with Parkinson’s disease. Tyrosine treatment enhanced working memory, task switching, response inhibition, and other cognitive control abilities in young adults, particularly under stressful conditions (
24). Canine distemper encephalomyelitis in immature dogs is associated with behavioral changes which are related to cerebral dysfunction (
8). Dysfunction of the brain stem’s ascending reticular activating system has been linked to loss of consciousness andbehavioral abnormalities. However, the neurological symptoms are associated with the affected brain regions, primarily the cortical and subcortical areas (
25). Therefore, the lower L-tyrosine concentrations of the dogs with neurological form of CDV infection in the present study may be a result of the altered mental state caused by the CDV-infected brain.
It was demonstrated that L-phenylalanine causes a selective, substantial, and reversible reduction of ionotropic glutamate receptor function at excitatory synapses in rat/mouse hippocampus, and in cerebrocortical neuronal cultures (
26). The unique action of L-phenylalanine involves the simultaneous activation of three distinct mechanisms that collectively contribute to the depression of glutamatergic synaptic transmission: (
1) competition for the glycine-binding site of N-methyl-D-aspartate receptors; (
2) competition for the glutamate-binding site of alpha-amino- 3-hydroxy 5-methylisoxazole-4-propionic acid/kainite receptors; and (
3) attenuation of glutamate release. Furthermore, results from studies using neuronal cultures or patients with phenylketonuria imply that transient elevations in L-phenylalanine concentration are unlikely to cause long-term alterations in brain and neuronal function. Moreover, it was indicated that L-phenylalanine can exert major neuroprotective actions via its derivates’ inhibitory effects on glutamatergic synaptic transmission (
27). Considering the role of the glutamatergic system in cell death and CDV causing massive cell death of neurons, low L-phenylalanine concentrations of the dogs with CDV infection may be associated with an inadequate neuroprotective response (
9).
Threonine plays multiple vital functions in the body, i.e., tissue protein synthesis, mucin production in enterocytes (lubrication and defense against pathogens), synthesis of collagen, elastin, tooth enamel, and glycine. As an indispensable amino acid, it must be obtained from the diet. Threonine is closely linked to glycine and serine through biochemical processes and membrane transporters. The Asc1 transporter facilitates the transport of D-serine, L-serine, L-alanine, L-threonine, and L-glycine across the neuronal membrane (
28). It was reported that threonine consumption and its levels in the plasma, brain stem and cortex are significantly correlated with the threonine and glycine cortical concentrations. Threonine was reported to be elevated in the CSF of patients with Alzheimer’s disease. Threonine, along with serine, is the potential precursor of glycine which is an inhibitory neurotransmitter. The glycine receptors are primarily located in the ventral spinal cord. Patients with Alzheimer’s disease may have higher levels of serine and threonine in their CSF as a result of tau protein breakdown (
29). Due to the neurotropic nature of CDV, decreased CSF L-threonin concentrations might be due to neurodegeneration of meningeal cells, neurons, granular cells, and Purkinje’s cells which contain tau proteins (
30).
Glycolysis in glial cells produces alanine which undergoes a reverse conversion into pyruvate within cholinergic neurons located in the olfactory memory center. This process aids the increased mitochondrial requirements of these neurons. Consequently, L-alanine derived from the glial glucose metabolism can function as a metabolic substrate and as alternative energy source to lactate (
31). Low glucose levels were reported in canine viral diseases including Canine Parvovirus and CDV. Non-fatal high viral titer may also cause relative hypoglycemia (
32). The low L-alanine CSF concentrations in dogs with CDV in the present study may be a result of impaired energy metabolism as a natural response to prevent viral replication resulting in cellular stress (
8).
Histidine is a necessary amino acid in dogs involved in several key metabolic processes, including the histaminergic system in the central nervous system. Human cases of juvenile myoclonic epilepsy and acute tonic-clonic seizures have both been linked to decreased histidine concentrations (
33). Histidine injections administered intraperitoneally have demonstrated a dose-dependent inhibition of chemically induced myoclonic jerks and tonic–clonic seizures in rats. The observed increase in histamine concentrations in the cerebral cortex, amygdala, and hippocampus of rats following histidine injections has led to the suspicion that the seizureinhibiting effect of histidine may be attributed to enhanced histamine formation (
34). Also, in individuals with Alzheimer’s disease, higher L-histidine CSF concentrations were reported. The high L-histidine CSF concentrations in the CDV-dogs from the current study could reflect the CDV-induced degenerative process in the brain. The ventricular CSF is a repository of amino acids from the brain (
29).
All cells require L-tryptophan for protein synthesis, and is acquired by food. Tryptophan metabolism produces a number of physiologically active compounds, including numerous significant neuromodulators of CNS activity. The correlation between total and free tryptophan plasma concentrations and its uptake rate in the brain is disputable. However, it is indicative that the tryptophan dissociation from albumins in the surrounding milieu of the cerebral microvascular system can enhance this. Tryptophan availability to the brain appears to be significantly regulated by sympathetic nervous system activation. The activation of the sympathetic nervous system can increase brain tryptophan concentrations following stress and immune-related responses in experimental models (
35). Viral infections activate the sympathetic nervous system leading to the release of norepinephrine which exerts proinflammatory effects by increasing macrophagederived TNF-α production via α2 adrenoceptors. Hence, higher CSF L-tryptophan concentrations detected in the dogs with CDV of the present study may be associated with sympathetic nervous system activation (
36).
The current study limitations are: not conducting Principal Component Analysis (PCA), Orthogonal Projections to Latent Structures (OPLS) analysis, and metabolite network mapping analysis for modeling spectroscopic data. Additionally, the study did not use PCR analysis for CDV diagnosis, CSF cytology, and protein analysis.
CONCLUSIONThe CSF samples in dogs with neurological CDV infection had significant differences in 1H-NMRbased amino acid profile compared to the Healthy group. Amino acid CSF concentrations could be used as markers for: L-tyrosine - assessing mental status changes, L-phenylalanine - neuroprotective responses, L-threonine and L-histidine - gauging the extent of neurodegeneration and ventricular degeneration, L-alanine - exploring cellular stress and energy metabolism, and L-tryptophan - understanding the process of sympathetic nervous system activation could hold potential clinical significance. Still, studies mapping the altered pathways of the identified amino acids are needed.
CONFLICT OF INTERESTThe authors declare that they have no known conflict of interest in the conduction of the current study.
ACKNOWLEDGMENTSThe research was supported by the Veterinary Faculty at the Harran University in Şanliurfa and the Veterinary Faculty at the Selçuk University in Konya.
AUTHORS’ CONTRIBUTIONEG, CB, İG, AŞ and MO developed the study design and concept, conducted the analyses and wrote the manuscript. EK and FA collected the samples. EG, CB and MO were involved in interpretation of data. All authors have revised and approved the final version of the manuscript.